Darwin’s Dilemma: The Origin and Evolution of the Eye
Published October 1, 2019
By Carl Zimmer
Academy Contributor
Award-winning science writer Carl Zimmer explains the “creation” of the organ so complex that it baffled even Darwin.
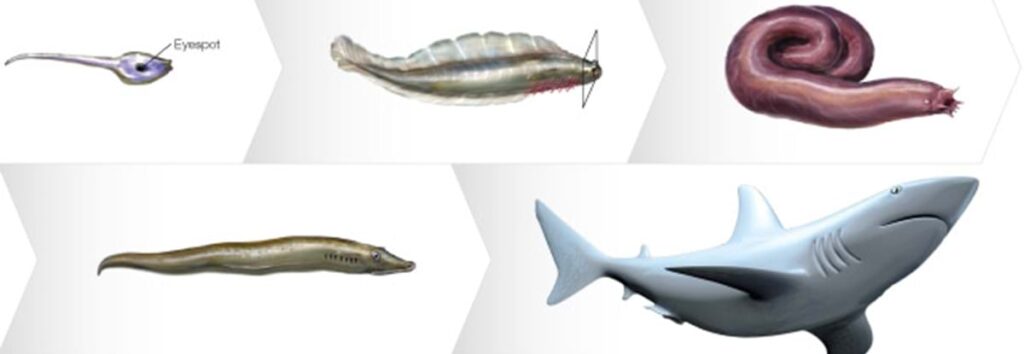
“The eye to this day gives me a cold shudder,” Charles Darwin once wrote to a friend.
If his theory of evolution was everything he thought it was, a complex organ such as the human eye could not lie beyond its reach. And no one appreciated the beautiful construction of the eye more than Darwin—from the way the lens was perfectly positioned to focus light onto the retina to the way the iris adjusted the amount of light that could enter the eye. In The Origin of Species, Darwin wrote that the idea of natural selection producing the eye “seems, I freely confess, absurd in the highest possible degree.”
For Darwin, the key word in that sentence was seems. If you look at the different sort of eyes out in the natural world and consider the ways in which they could have evolved, Darwin realized, the absurdity disappears. The objection that the human eye couldn’t possibly have evolved, he wrote, “can hardly be considered real.”
Dozens of Different Kinds of Eyes
Today evolutionary biologists are deciphering the origins of not just our own eyes but the dozens of different kinds of eyes that animals use. Fly eyes are built out of columns. Scallops have a delicate chain of eyes peeking out from their shells. Flatworms have simple light-sensitive spots. Octopuses and squids have camera eyes like we do, but with some major differences. The photoreceptors of octopuses and squids point out from the retina, towards the pupil. Our own eyes have the reverse arrangement. Our photoreceptors are pointed back at the wall of the retina, away from the pupil.
For decades, most scientists argued that these different eyes evolved independently. The earliest animals that lived over 600 million years ago were thought to be eyeless creatures. As their descendants branched out into different lineages, some of them evolved their own kinds of eyes. It now turns out, however, that this is not really true.
All eyes, in all their wonderful variety, share an underlying unity in the genes used to build them. By tracing the history of these shared genes, scientists uncovering the steps by which complex eyes have evolved through a series of intermediate steps.
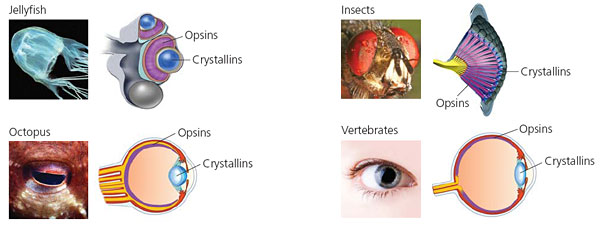
Opsins in Common
When light enters your eye, it strikes a molecule known as an opsin. Opsins sit on the surface of photoreceptor cells, and when they catch photons, they trigger a series of chemical reactions that causes the photoreceptor to send an electrical message towards the brain.
Biologists have long known that all vertebrates carry the same basic kind of opsin in their eyes, known as a c-opsin. All c-opsins have the same basic molecular shape, whether they’re in the eye of a shark or the eye of a hummingbird. All c-opsins are stored in a stack of disks, each of which grows out of a hair-like extension of the retina called a cilium.
In all vertebrates, c-opsins relay their signal from the stack of disks through a pathway of proteins called the phosphodiesterase pathway. All of these homologies suggest that c-opsins were present in the common ancestor of all living vertebrates.
Vertebrates belong to a much larger group of species known as bilaterians—in other words, animals that develop a left-right symmetry. The main lineage of these other bilaterians, known as protostomes, includes millions of species, ranging from insects to earthworms and squid.
Protostome eyes don’t have the c-opsins found in vertebrates. Instead, protostomes build another molecule, known as an r-opsin. Instead of keeping r-opsins in a stack of disks, they store r-opsins in foldings in the membranes of photoreceptors. R-opsins all send their signals through the same pathway of proteins (not the same pathway as c-opsins send signals in vertebrates).
Humans Also Make R-Opsins
These similarities in the r-opsins suggest they evolved in the common ancestor of protostomes, only after their ancestors had branched off from the ancestors of vertebrates. Likewise, vertebrates only evolved c-opsins in their eyes after the split. In recent years, however, evolutionary biologists have discovered opsins where they weren’t supposed to be.
It turns out, for example, that humans also make r-opsins. We just don’t make them on the surfaces of photoreceptors where they can catch light. Instead, r-opsins help to process images captured by the retina before they’re transmitted to the brain.
In 2004, Detlev Arendt of the European Molecular Biology Laboratory and his colleagues also found c-opsins where they weren’t supposed to be. They were probing the nervous system of an animal known as a ragworm, which captures light with r-opsins. Arendt and his colleagues discovered a pair of organs atop the ragworm’s brain that grew photoreceptors packed with c-opsins.
Arendt sequenced the gene for the ragworm c-opsins and compared it with genes for other opsins. He found that it is more closely related to the genes for c-opsins in our own eyes than it is to the genes for r-opsins in the ragworm’s own eyes. These findings have led Arendt and other researchers to revise their hypothesis about the origin of opsins: the common ancestor of all bilaterians must already have had both kinds of opsins.
Clues from Cnidarians
But Todd Oakley, a biologist at the University of California at Santa Barbara, wondered if opsins might be even older. To find out, Oakley and his colleagues turned to the closest living relatives of bilaterians. Known as the cnidarians, this lineage includes jellyfish, sea anemone, and corals.
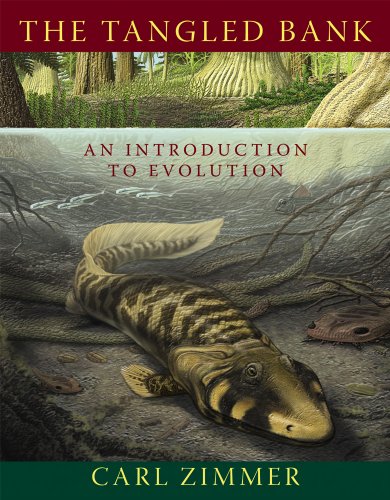
Biologists have long known that some cnidarians can sense light. Some jellyfish even have eye-like organs that can form crude images. In other ways, though, cnidarians are radically different from bilaterians. They have no brain or even a central nerve cord, for example. Instead, they have only a loose net of nerves. These dramatic differences had led some researchers to hypothesize that bilaterians and cnidarians had evolved eyes independently. In other words, the common ancestor of cnidarians and bilaterians did not have eyes.
In recent years, scientists have sequenced the entire genomes of two species of cnidarians, the stellar sea anemone (Nematostella vectensis) and a freshwater hydra (Hydra magnipapillata). Scanning their genomes, Oakley and his colleagues discovered that both species cnidarians have genes for opsins—the first time opsin genes had ever been found in a nonbilaterian. The scientists carried out experiments on some of these genes and discovered that they are expressed in the sensory neurons of the cnidarians. Oakley’s research suggests that, as he had suspected, opsins evolved much earlier than bilaterians.
How Opsins Evolved
With discoveries from scientists such as Oakley and Arendt, we can start to get a sense of how opsins evolved. Opsins belong to a family of proteins called G-protein coupled receptors (GPCRs). They’re also known as serpentine proteins, for the way they snake in and out of cell membranes. Serpentine proteins relay many different kinds of signals in the cells of eukaryotes. Yeast cells use them to detect odorlike molecules called pheromones released by other yeast cells. Early in the evolution of animals, a serpentine protein mutated so that it picks up a new kind of signal: light.
At some point, the original opsin gene was duplicated (Figure 8.13). The two kinds of opsins may have carried out different tasks. One may have been sensitive to a certain wavelength of light, for example, while the other tracked the cycle of night and day. When cnidarians and bilaterians diverged, perhaps 620 million years ago, they each inherited both kinds of opsins. In each lineage, the opsins were further duplicated and evolved into new forms. And thus, from a single opsin early in the history of animals, a diversity of light-sensing molecules has evolved.
The Crystalline Connection
The earliest eyes were probably just simple eyespots that could only tell the difference between light and dark. Only later did some animals evolve spherical eyes that could focus light into images. Crucial to these image-forming eyes was the evolution of lenses that could focus light. Lenses are made of remarkable molecules called crystallins, which are among the most specialized proteins in the body. They are transparent, and yet can alter the path of incoming light so as to focus an image on the retina. Crystallins are also the most stable proteins in the body, keeping their structure for decades. (Cataracts are caused by crystallins clumping late in life.)
It turns out that crystallins also evolved from recruited genes. All vertebrates, for example, have crystallins in their lenses known as α-crystallins. They started out not as light-focusing molecules, however, but as a kind of first aid for cells. When cells get hot, their proteins lose their shape. They use so-called heat-shock proteins to cradle overheated proteins so that they can still carry out their jobs.
Scientists have found that α-crystallins not only serve to focus light in the eye, but also act as heat-shock proteins in other parts of the body. This evidence indicates that in an early vertebrate, a mutation caused α-crystallins to be produced on the surface of their eyes. It turned out to have the right optical properties for bending light. Later mutations fine-tuned α-crystallins, making them better at their new job.
The Evolution of the Vertebrate Eye
Vertebrates also produce other crystallins in their eyes, and some crystallins are limited to only certain groups, such as birds or lizards. And invertebrates with eyes, such as insects and squid, make crystallins of their own. Scientists are gradually discovering the origins of all these crystallins. It turns out that many different kinds of proteins have been recruited, and they all proved to be good for bending light.
In 2007, Trevor Lamb and his colleagues at Australian National University synthesized these studies and many others to produce a detailed hypothesis about the evolution of the vertebrate eye. The forerunners of vertebrates produced light-sensitive eyespots on their brains that were packed with photoreceptors carrying c-opsins. These light-sensitive regions ballooned out to either side of the head, and later evolved an inward folding to form a cup.
Early vertebrates could then do more than merely detect light: they could get clues about where the light was coming from. The ancestors of hagfish branched off at this stage of vertebrate eye evolution, and today their eyes offer some clues to what the eyes of our own early ancestors would have looked like.
The Evolution Doesn’t Stop
After hagfish diverged from the other vertebrates, Lamb and his colleagues argue, a thin patch of tissue evolved on the surface of the eye. Light could pass through the patch, and crystallins were recruited into it, leading to the evolution of a lens. At first the lens probably only focused light crudely. But even a crude image was better than none. A predator could follow the fuzzy outline of its prey, and its prey could flee at the fuzzy sight of its attackers. Mutations that improved the focusing power of the lens were favored by natural selection, leading to the evolution of a spherical eye that could produce a crisp image.
The evolution of the vertebrate eye did not stop there. Some evolved the ability to see in the ultraviolet. Some species of fish evolved double lenses, which allowed them to see above and below the water’s surface at the same time. Vertebrates adapted to seeing at night and in the harsh light of the desert. Salamanders crept into caves and ended up with tiny vestiges of eyes covered over by skin. But all those vertebrate eyes were variations on the same basic theme established half a billion years ago.
About the Author
Carl Zimmer is a lecturer at Yale University, where he teaches writing about science and the environment. He is also the first Visiting Scholar at the Science, Health, and Environment Reporting Program at New York University’s Arthur L. Carter Journalism Institute.
Zimmer’s work has been anthologized in both The Best American Science Writing series and The Best American Science and Nature Writing series. He has won numerous fellowships, honors, and awards, including the 2007 National Academies Science Communication Award for “his diverse and consistently interesting coverage of evolution and unexpected biology.”
His books include Soul Made Flesh, a history of the brain; Evolution: The Triumph of an Idea; At the Water’s Edge, a book about major transitions in the history of life; The Smithsonian Intimate Guide to Human Origins; and Parasite Rex, which the Los Angeles Times described as “a book capable of changing how we see the world.”
His newest book, The Tangled Bank: An Introduction to Evolution, will be published this fall to coincide with the 150th anniversary of the publication of The Origin of Species.