The Genius of Quantum Physicist Richard Feynman
Missives from Feynman in Perfectly Reasonable Deviations from the Beaten Track, a book of his letters edited by daughter Michelle Feynman, reveal his genius and wit. What was his contribution to the canon of 20th-century quantum physics?
Published February 3, 2006
By Chris H. Greene
Academy Contributor
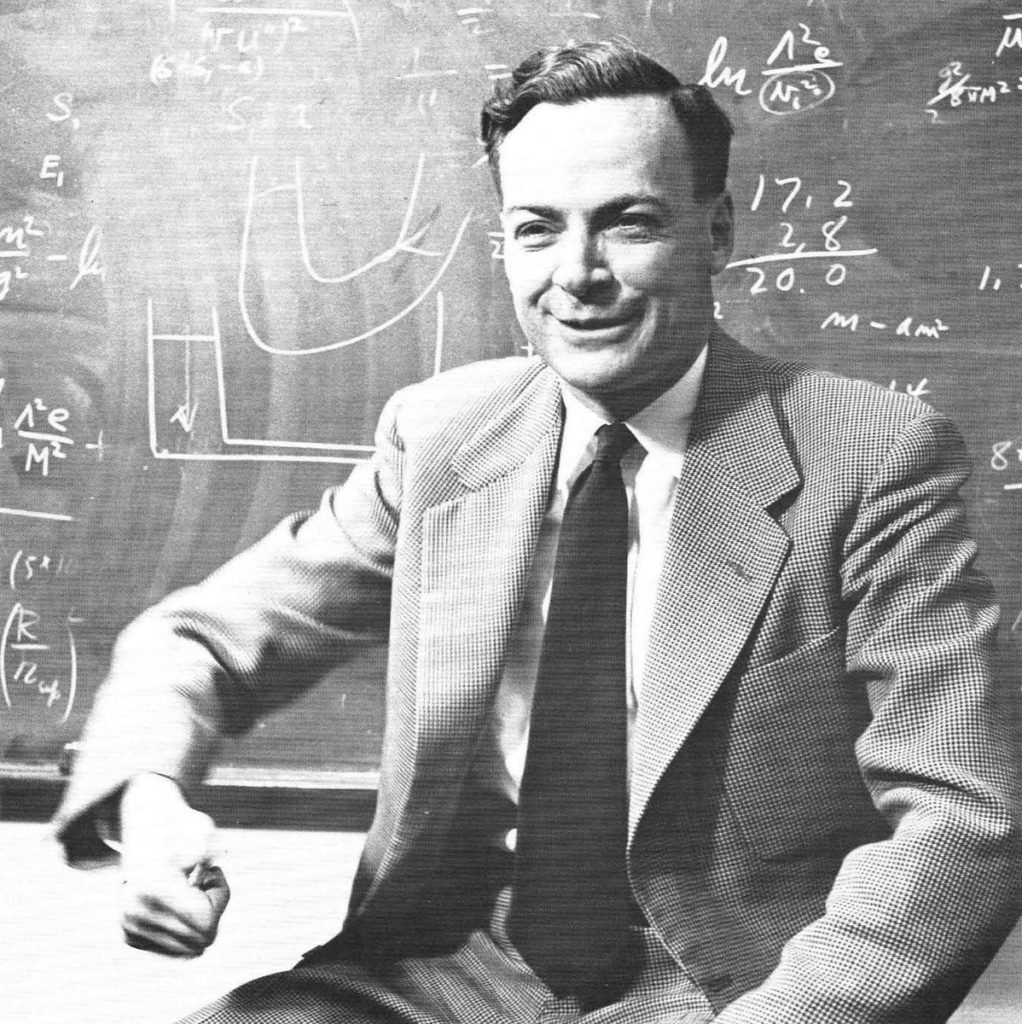
“Science alone of all the subjects contains within itself the lesson of the danger of belief in the infallibility of the greatest teachers in the preceding generation … Learn from science that you must doubt the experts. As a matter of fact, I can also define science another way: Science is the belief in the ignorance of experts.”
— Richard Feynman, 1981
We all know the stories of Richard Feynman. He was at times a showman and a clown. He expressed irreverence toward prestigious, hoary organizations like the National Academy of Sciences and the Royal Swedish Academy of Sciences. The tragic death of his young wife during the time of the Manhattan Project became familiar to millions through the touching Matthew Broderick film, Infinity. But behind his public persona lay one of the truly independent and innovative minds of the 20th century. Richard Feynman felt an intense, personal need to see physical phenomena in his own terms, and from his own perspectives, using theories that he generated himself.
At the same time, Feynman’s theoretical constructs did not arrive on the planet like a bolt from nowhere. His most important contributions were ideas that were in some sense already “in the wind,” but his way of developing them into consistent theoretical descriptions of nature differed dramatically from methods popular at the time.
Paradoxical Infinities
It may seem surprising, but the theoretical program that resulted in Feynman’s 1965 Nobel Prize (also awarded that year to Julian Schwinger and Sin-Itiro Tomonaga) was not aimed so much at explaining the result of any particular experiment, as it was an attempt to resolve some of the apparently self-contradictory aspects of both classical and quantum electrodynamics theory. If you shake an electron, it radiates light waves, whose electric fields must in turn act back on the electron to lower its energy. But attempts to calculate this “radiative reaction force” led to infinities which were paradoxical and in clear contradiction with experience.
In Feynman’s doctoral thesis work with John Wheeler at Princeton, the two entertained fantastic possibilities in a desperate attempt to solve these paradoxical infinities. One peculiar notion that emerged was that if, in a certain sense, the classical fields are allowed to propagate backward in time, the paradoxes and the infinities appeared to be magically removed.
A variant of this idea survived when Feynman wrote down his quantum mechanical formulation of this problem, which he credits to Wheeler for originally tossing out: that the positron, the antiparticle of an electron, can be regarded as an ordinary electron moving backward in time. Surely you’re joking, Mr. Feynman! As fantastic and unbelievable as this idea seems when stated in words, when formulated mathematically it was found that a consistent theoretical framework emerged, without the troubling infinities.
Moreover, Feynman created a simple way for these complicated calculations to be carried out, which is still used today: first, draw lines that represent electrons, positrons, and photons moving forward and backward in time in different ways that can contribute to the process of interest. Then apply Feynman’s rules for translating each such Feynman diagram into a precise mathematical formula.
Quantum Electrodynamics
One of the most famous applications of Feynman’s quantum electrodynamics was his calculation of a tiny frequency difference between two nearly identical energy levels (2S1/2 and 2P1/2) of the simplest atom, hydrogen. Willis Lamb and Robert C. Retherford had caused a stir in 1947 when they measured this frequency difference to be 1057 million cycles per second (MHz), because the then-accepted theory of Paul Dirac suggested that this difference should be identically zero. The methods for calculating this interaction between an atomic electron and the “vacuum-fluctuating electric fields of free space” gave infinity, a useless result entirely irrelevant to the experiment.
Using the Feynman calculus, however, a result very close to the experimental frequency splitting (the so-called “Lamb shift”) was obtained. In the intervening decades, both experiment and theory have improved, and we now know this Lamb shift experimentally to be 1057.8447 (plus or minus 0.0034) MHz, while theory based on Feynman’s work predicts 1057.839 (plus or minus 0.006) MHz.
Within experimental uncertainties, and within theoretical uncertainties associated with our imperfect understanding of the proton’s nuclear structure, these agree. Nature thus confirms the remarkable synthesis of theoretical ideas into working quantum electrodynamics, achieved by Feynman, as well as by Schwinger and by Tomonaga.
Advancing the World of Theoretical Physics
And what are we to take from these strange notions? Are positrons really just electrons moving backward in time? Feynman tended to dismiss such queries as having no more relevance to physics than debates about how many angels fit on the head of a pin. Here is one more example where the equations developed by theoretical physicists, after extensive testing, are the bottom line. Seemingly bizarre philosophical implications, when those equations are stated in words (such as “particles moving backward in time”), do not matter a whit. What matters from the physicist’s perspective is the explanatory and predictive power of the resulting theory.
In the end, Feynman’s work parallels eerily the way the “luminiferous aether” was abandoned as irrelevant, once physicists accepted around the beginning of the 20th century that Maxwell’s equations by themselves adequately describe all classical phenomena of electricity and magnetism. And it is similar to the way Einstein’s equations of relativity, and the peculiar quantum theory, were accepted despite their troubling, almost nonsensical implications for how we think about time, space, and reality. As Niels Bohr wrote and was quoted in Wheeler and Feynman’s 1945 Reviews of Modern Physics article:
We must, therefore, be prepared to find that further advance…will require a still more extensive renunciation of features which we are accustomed to demand of the space time mode of description.
The world of theoretical physics is better today because Richard Feynman was brave enough to contemplate and develop ideas that required such a renunciation.
Also read: The Challenge of Quantum Error Correction