Innovative Vaccines: Tackling Infectious Diseases and Emerging Threats
Reported by:
Rebecca Vaadia
Presented by:
Microbiology and Infectious Diseases Discussion Group
The New York Academy of Sciences
Overview
While the development of vaccines against infectious diseases has had a profound impact on life expectancy, there remain many resistant and emerging infections for which no effective vaccines are available, such as malaria, HIV, and Zika. Recent advances in biotechnology and our understanding of human immunity hold great promise for conquering new diseases. For example, advances in structural biology allow for the discovery of new antigens that can target broad viral families, such as influenza, or complex parasites like malaria. Novel clinical trials for maternal immunizations have shown encouraging results for reducing dangerous diseases in newborn infants. Furthermore, recent progress in DNA- or RNA-based vaccines holds promise for inexpensive and fast production, which is especially favorable for responding to emerging epidemics. Learn more about recent breakthroughs in vaccine development in this summary of our May 20, 2019 symposium, which gathered the world’s leaders in vaccine development.
Symposium Highlights:
- Emerging infectious diseases can be treated quickly with a passive vaccine containing human monoclonal antibodies isolated from the blood of an infected patient.
- Targeting multiple stages of the malaria life cycle is a promising strategy for the development of a successful vaccine targeting this complex parasite.
- Clinical trials show promise for maternal immunizations in protecting newborn infants from respiratory syncytial virus (RSV) and Group B streptococcus.
- A vaccine containing the influenza hemagglutinin (HA) fusion protein without the head domain can elicit protection against a broad group of influenza viruses.
- Synthetic DNA and mRNA vaccines are simple to manufacture and show promise for treating a wide range of diseases, including Ebola, HIV, Zika, influenza, and malaria.
- A promising new adjuvant, AS01, has contributed to breakthrough vaccines for Malaria, tuberculosis, and shingles.
Speakers
James E. Crowe, Jr., MD
Vanderbilt University Medical Center
Greg Glenn, MD
Novavax
Adrian Hill, PhD
University of Oxford
Kathrin Jansen, PhD
Pfizer
Wayne Koff, PhD
Human Vaccines Project
Sallie Permar, MD, PhD
Duke University
Rino Rappuoli, PhD
GlaxoSmithKline
David Weiner, PhD
The Wistar Institute
Drew Weissman, MD, PhD
University of Pennsylvania
Ian Wilson, DPhil
The Scripps Research Institute
Event Sponsors
Silver
Bronze
Academy Friend
New Approaches for Understanding the Immune System for Vaccine Development
Speaker
James E. Crowe, Jr.
Vanderbilt University Medical Center
Human Antibodies and Repertoires for Emerging Infectious Diseases
James Crowe, of Vanderbilt University Medical Center, discussed his lab’s work developing treatments for emerging infectious diseases using monoclonal human antibodies. “Antibodies essentially are a passive vaccine,” explained Crowe. Currently, it takes about two years to develop a vaccine for an infectious disease agent, which is not quick enough for outbreak response. Therefore, Crowe argues that antibodies are the “most appropriate public health measure for most emerging infections.” Crowe’s group is working on two strategies for developing human antibody drugs: one focuses on speed, whereas the other aims to develop broad antibodies ahead of an outbreak.
The Rapid Rational Antibody Design and Delivery (RRADD) project uses ultra-fast techniques to respond to a specific outbreak in the moment. They recently used Zika as a test case. Starting with a blood sample from a surviving patient, their facility used single-cell RNA-sequencing to produce a list of antibody genes within a day. These antibodies were quickly produced and then tested in a high-throughput real-time cell culture system to assay for protection against Zika infection. Leading candidates were tested in mouse and primate models, leading to the discovery of protective antibodies within 78 days.
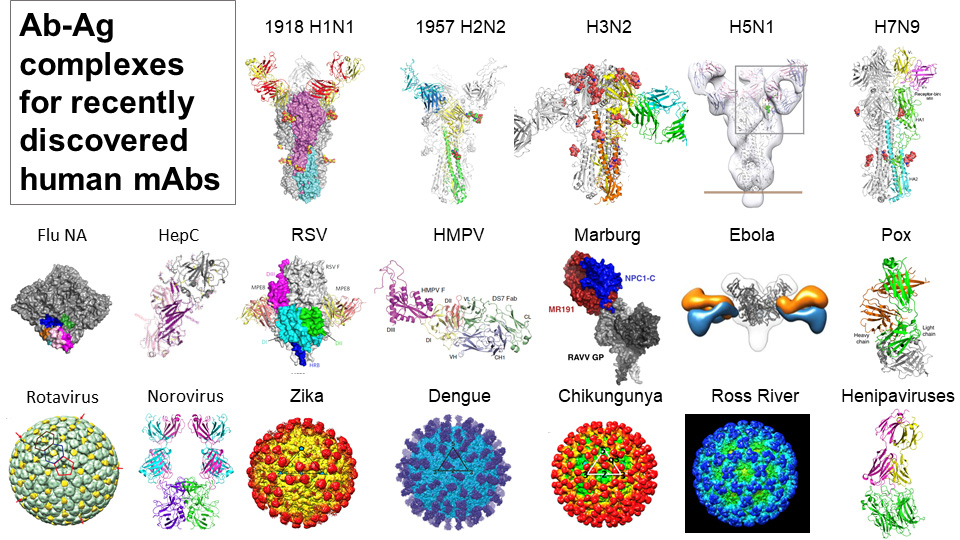
The second strategy is the Advanced Human Epidemic Antibody Defenses (AHEAD100) project, a methodical approach that aims to develop antibodies for the 100 most likely infectious diseases ahead of any future outbreaks. Interestingly, they found broad antibodies that work across viruses of a related class, such as noroviruses, alphaviruses, and flu.
Further Readings
Crowe
Bangaru S, Lang S, Schotsaert M, et al.
A Site of Vulnerability on the Influenza Virus Hemagglutinin Head Domain Trimer Interface.
Cell. 2019;177(5):1136-1152 e1118.
Finn JA, Crowe JE, Jr.
Impact of new sequencing technologies on studies of the human B cell repertoire.
Curr Opin Immunol. 2013;25(5):613-618.
Flyak AI, Ilinykh PA, Murin CD, et al.
Mechanism of human antibody-mediated neutralization of Marburg virus.
Cell. 2015;160(5):893-903.
Hasan SS, Miller A, Sapparapu G, et al.
A human antibody against Zika virus crosslinks the E protein to prevent infection.
Nat Commun. 2017;8:14722.
Sapparapu G, Fernandez E, Kose N, et al.
Neutralizing human antibodies prevent Zika virus replication and fetal disease in mice.
Nature. 2016;540(7633):443-447.
Wang H, Shi Y, Song J, et al.
Ebola Viral Glycoprotein Bound to Its Endosomal Receptor Niemann-Pick C1.
Cell. 2016;164(1-2):258-268.
Taking on the Big Challenges Facing Novel Vaccine Development
Adrian Hill
University of Oxford
Wayne Koff
Human Vaccines Project
New Generation Malaria Vaccines
Adrian Hill from the University of Oxford presented his work on the development of a malaria vaccine. Malaria causes 500,000 deaths each year, but developing an effective vaccine is challenging. “Even if you get a good antigen, you need remarkably high immunogenicity,” Hill explained. Therefore, Hill’s group aims to develop a vaccine that targets multiple stages of the malaria parasite life cycle.
In the first stage, mosquitos introduce malaria sporozoites into a human host. Hill’s group and others have been developing vaccines that combine malaria antigens with virus-like particles to induce antibody production against sporozoites. Hill and colleagues are developing R21, a more potent version of the RTS,S vaccine currently in Phase III trials. In R21, 100% of the molecules encode the sporozoite antigen. Studies show that this formulation allows for a lower dose, as antibody titers are indistinguishable between a 10 µg dose of R21 and a 50 µg dose of RTS,S. Furthermore, R21 shows a more durable response, with higher titers at six months versus RTS,S. By 2020, they expect efficacy results from the first Phase IIB trial.
As the malaria life cycle progresses, sporozoites infect liver cells, where the parasite matures. “[To target] the liver stage, you need T-cells” said Hill. Inducing T-cells requires a viral vector approach. Research on mice and clinical studies from Hill’s group show that the ME-TRAP antigen viral vector can induce high levels of resident memory T-cells in the liver. There are ongoing field clinical trials for this vaccine.
The Future of Vaccine Development
Wayne Koff, the president and CEO of the Human Vaccines Project, described the nonprofit’s research decoding the human immune system. Vaccines for complex infectious and non-communicable diseases such as HIV, tuberculosis, and cancer have been difficult to develop. Koff believes that a better understanding of human immunity is essential for accelerating vaccine development for these diseases.
One strategy is to investigate why some people respond to vaccines and infections much better than others. “If we can understand this, we can get at the pathogens we haven’t been able to tackle,” said Koff. Recent developments in single cell multi-omics allow for an in-depth analysis of an individual’s immune system. A growing body of evidence suggests that immunity biomarkers at baseline can predict an individual’s response to immunization. Researchers performed single cell RNA-sequencing on innate immune cells before immunization and successfully identified biomarkers predictive of the response to the Hepatitis B vaccine. By integrating all of the pre-immunization data, investigators could build biostatistical models that accurately predicted final antibody titers, while revealing pathways that may be involved in the response mechanism.
This data suggests that “we all have an immune set point,” said Koff, which leads to the opportunity to modulate this set point before immunization to improve outcomes. Furthermore, smaller trials that account for individual variability and assay predictive signatures may be more effective than standard large vaccine efficacy trials.
Further Readings
Hill
Gola A, Silman D, Walters AA, et al.
Prime and target immunization protects against liver-stage malaria in mice.
Sci Transl Med. 2018;10(460).
Greenwood B, Doumbo OK.
Implementation of the malaria candidate vaccine RTS,S/AS01.
Lancet. 2016;387(10016):318-319.
Klein SL, Shann F, Moss WJ, Benn CS, Aaby P.
RTS,S Malaria Vaccine and Increased Mortality in Girls.
MBio. 2016;7(2):e00514-00516.
Ogwang C, Kimani D, Edwards NJ, et al.
Sci Transl Med. 2015;7(286):286re285.
Koff
Antia A, Ahmed H, Handel A, et al.
Heterogeneity and longevity of antibody memory to viruses and vaccines.
PLoS Biol. 2018;16(8):e2006601.
Briney B, Inderbitzin A, Joyce C, Burton DR.
Commonality despite exceptional diversity in the baseline human antibody repertoire.
Nature. 2019;566(7744):393-397.
Fourati S, Cristescu R, Loboda A, et al.
Nat Commun. 2016;7:10369.
Liu X, Speranza E, Munoz-Fontela C, et al.
Genome Biol. 2017;18(1):4.
Soto C, Bombardi RG, Branchizio A, et al.
High frequency of shared clonotypes in human B cell receptor repertoires.
Nature. 2019;566(7744):398-402.
Team H-CSP, Consortium H-I.
Multicohort analysis reveals baseline transcriptional predictors of influenza vaccination responses.
Sci Immunol. 2017;2(14).
Tsang JS, Schwartzberg PL, Kotliarov Y, et al.
Global analyses of human immune variation reveal baseline predictors of postvaccination responses.
Cell. 2014;157(2):499-513.
Vaccines to Protect Newborns: The Next Frontier
Speakers
Greg Glenn
Novavax
Kathrin Jansen
Pfizer
Sallie Permar
Duke University
Advances in Maternal Immunization
Kathrin Jansen from Pfizer discussed recent advances in maternal immunization. Infants under six months are the most vulnerable to infection, but most vaccines are not available at this early stage of life. Furthermore, “20% of stillbirths seem to be associated with an infectious disease,” said Jansen. Active antibody transfer from mother to baby during pregnancy is an essential mechanism for protecting infants from infectious diseases. The goal of maternal immunization is to enhance maternal antibody levels to further protect newborns. Jansen explained that these vaccines could either “augment pre-existing antibody responses or induce a de novo response” to infections the mother has not yet been exposed to.
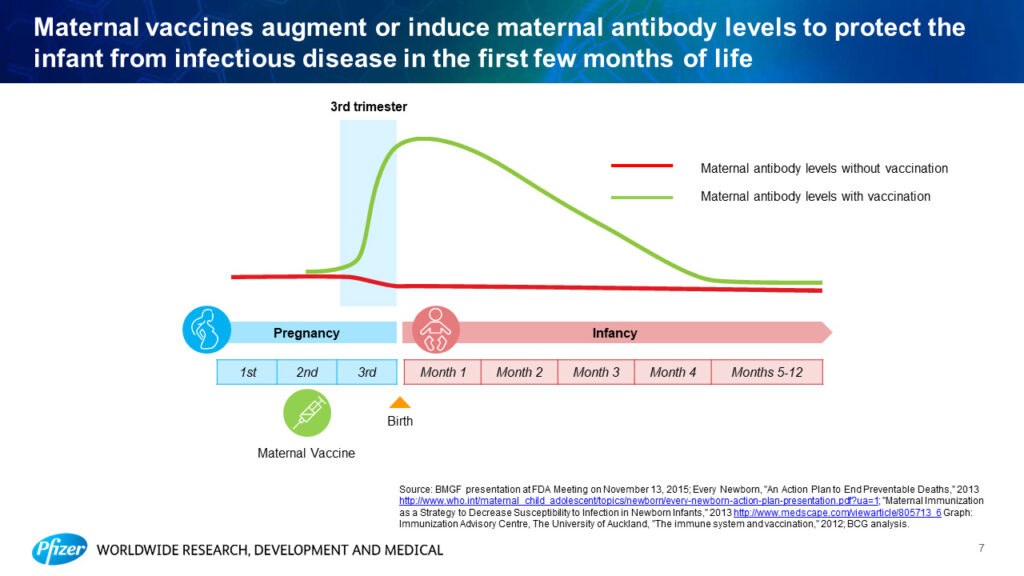
Jansen presented recent findings for maternal vaccines targeting respiratory syncytial virus (RSV) and Group B streptococcus bacteria, two infections that are especially deadly for newborn infants. In a recent Phase I/II trial, the Group B streptococcus vaccine induced high levels of antibody titers for up to six months in healthy adults, giving confidence to move forward for testing in pregnancy. Recent structural biology studies of RSV identified a metastable form of the viral fusion protein. With this form in mind, a screen for vaccine candidates revealed molecules that were 30 times more powerful than the current licensed prophylactic antibody in rodents. Data from a Phase I/II study will be available later this year.
Protecting Infants from RSV via Maternal Immunization
Greg Glenn, of Novavax, presented recent progress on the development of an RSV maternal vaccine. RSV is the leading cause of hospitalization of infants in the United States. While the Pfizer version of the vaccine, described by Kathrin Jansen, resembles the metastable prefusion form of the viral fusion protein, the Novavax version targets an earlier, stable form known as the prefusogenic form. This vaccine contains a near full-length fusion protein, but with deletions in a furin cleavage site. “These deletions fix the protein structure, and that allows it to be very stable,” Glenn explained. Through stabilizing the prefusogenic form, the virus is prevented from successfully infecting cells, which allows the vaccine to be produced in culture with higher yields. Furthermore, all antibodies that target the metastable prefusion form also target the prefusogenic form. Immunization with the Novavax vaccine induces antibodies to a variety of viral epitopes, which are also transferred to the infant.
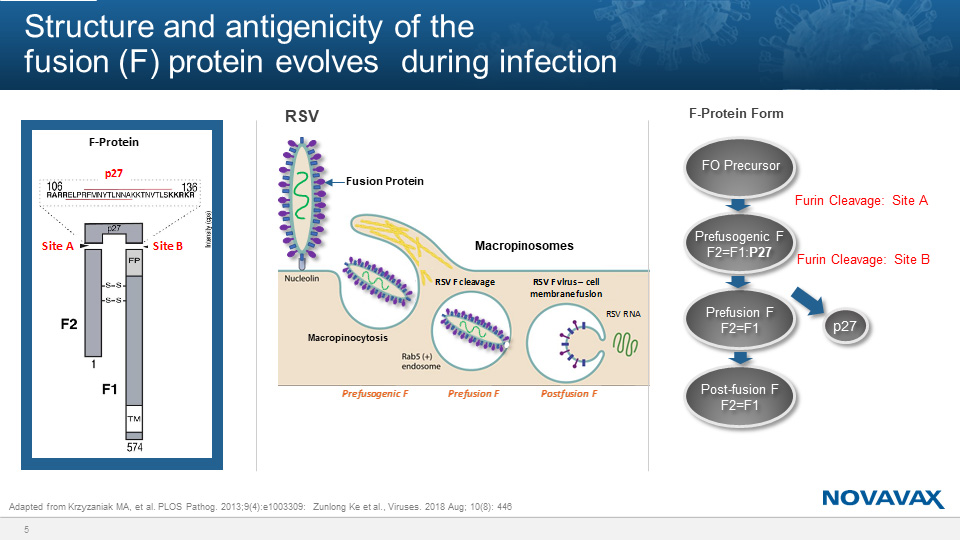
Currently, Novavax is running a worldwide Phase III randomized placebo-controlled trial to evaluate protection of infants against RSV with their maternal vaccine. The vaccine was given “to immunized mothers in third trimester, and we monitored infants intensely for six months,” explained Glenn. The trial showed a 40% reduction in their primary endpoint, which was medically significant RSV lower respiratory tract infection at 90 days old.
Next Generation Vaccines to Eliminate Congenital Cytomegalovirus: We are halfway there
Sallie Permar, of Duke University, shared her work developing an effective vaccine for congenital cytomegalovirus (CMV), which is the most common congenital infection and cause of birth defects worldwide. Developing a vaccine has been tricky, as it’s unknown exactly what maternal immune responses are protective against congenital CMV transmission. Permar’s group is investigating these questions with a novel, non-human primate model as well as data analysis from previous vaccine trials.
Permar and colleagues infected seronegative rhesus monkeys with CMV at the beginning of pregnancy. “We used a model of severe pathology with maternal CD4+ T-cell depletion followed by an intravenous inoculation to ask whether antibodies alone could be protective against congenital CMV transmission,” explained Permar. Data from a small group of animals suggests that treatment with passive antibodies from donor plasma prior to inoculation prevents fetal transmission. This result indicates that stimulating potent antibody responses could be a promising route to an effective maternal CMV vaccine.
Previous trials of a vaccine containing glycoprotein B, the main fusion protein of the virus, have shown partial effectiveness. Permar’s group probed the trial data to investigate what immune responses correlate with protection against CMV in infected versus uninfected vaccine recipients. “The ability of vaccine-elicited antibodies to bind to glycoprotein B-transfected cells was higher in uninfected vaccinees,” said Permar, suggesting that eliciting antibodies that bind to glycoproteins is a promising vaccine target. Furthermore, the infected group of vaccine recipients was still protected against specific CMV strains, suggesting that a broader immunogen might be more effective.
Further Readings
Jansen
Lindsey B, Kampmann B, Jones C.
Maternal immunization as a strategy to decrease susceptibility to infection in newborn infants.
Curr Opin Infect Dis. 2013;26(3):248-253.
McClure EM, Garces A, Saleem S, et al.
BJOG. 2018;125(2):131-138.
Wilcox CR, Holder B, Jones CE.
Front Immunol. 2017;8:1294.
Zaman K, Roy E, Arifeen SE, et al.
Effectiveness of maternal influenza immunization in mothers and infants.
N Engl J Med. 2008;359(15):1555-1564.
Glenn
Ke Z, Dillard RS, Chirkova T, et al.
The Morphology and Assembly of Respiratory Syncytial Virus Revealed by Cryo-Electron Tomography.
Viruses. 2018;10(8).
Krzyzaniak MA, Zumstein MT, Gerez JA, Picotti P, Helenius A.
PLoS Pathog. 2013;9(4):e1003309.
Shi T, McAllister DA, O’Brien KL, et al.
Lancet. 2017;390(10098):946-958.
Permar
Baraniak I, Kropff B, Ambrose L, et al.
Proc Natl Acad Sci U S A. 2018;115(24):6273-6278.
Bernstein DI, Munoz FM, Callahan ST, et al.
Vaccine. 2016;34(3):313-319.
Bialas KM, Tanaka T, Tran D, et al.
Proc Natl Acad Sci U S A. 2015;112(44):13645-13650.
Nelson CS, Huffman T, Jenks JA, et al.
HCMV glycoprotein B subunit vaccine efficacy mediated by nonneutralizing antibody effector functions.
Proc Natl Acad Sci U S A. 2018;115(24):6267-6272.
Nelson CS, Vera Cruz D, Su M, et al.
J Virol. 2019;93(5).
Pass RF, Zhang C, Evans A, et al.
Vaccine prevention of maternal cytomegalovirus infection.
N Engl J Med. 2009;360(12):1191-1199.
Renzette N, Gibson L, Bhattacharjee B, et al.
Rapid intrahost evolution of human cytomegalovirus is shaped by demography and positive selection.
PLoS Genet. 2013;9(9):e1003735.
New Technologies for Vaccine Development
Speakers
Rino Rappuoli, PhD
GlaxoSmithKline
David Weiner, PhD
The Wistar Institute
Drew Weissman, MD, PhD
University of Pennsylvania
Ian Wilson, DPhil
The Scripps Research Institute
Guiding Vaccine Candidates: Antibodies That Can Neutralize Influenza and Malaria
Ian Wilson, from the Scripps Research Institute, shared his recent work investigating the structural biology of antibodies to guide vaccine candidates for influenza and malaria. Wilson’s group aims to “design immunogens or even small molecules from the structural information about how antibodies bind.”
Human antibodies that neutralize a broad range of flu subtypes have been characterized in the last ten years. Interestingly, the broadest antibodies bind to the less immunogenic “stem” domain of the influenza hemagglutinin (HA) fusion protein, rather than the “head” domain. “We are using this information to try to think of novel vaccines,” said Wilson. “If we chop off the immunogenic head, then we can target the response against the stem.” Indeed, a recently developed headless HA construct elicited protection against all influenza A group 1 antibodies in mice and monkeys.
Wilson’s group has also probed the structural biology of human antibodies elicited in recent RTS,S malaria vaccine trials. Cryo-EM revealed the structure of antibodies binding to the circumsporozoite protein (CSP) of malaria: the antibodies spiral all the way around the NANP peptide repeats of the protein. Furthermore, antibodies in the spiral bind in close proximity, and often, somatic mutations strengthen these homotypic contacts for a more stable spiral. Future work will explore the relevance of this spiral structure for vaccine purposes.
Synthetic DNA Approaches for Difficult Infectious Disease Targets
David Weiner, of the Wistar Institute, presented recent findings on the development and efficacy of synthetic DNA vaccines. DNA vaccines are “very consistent, very simple to manufacture, temperature stable,” and allow for local transfection without systemic expression, explained Weiner. Recent early stage clinical trials have shown promising results for using synthetic DNA vaccines as immunotherapy to treat human papillomavirus (HPV)-related cancers. Synthetic DNA is also promising for treating emerging infectious diseases. Wiener discussed three examples, Ebola, MERS, and Zika, where prophylactic treatment with synthetic DNA induced a 95%–100% response rate, and transmission into the clinic occurred in only 7–15 months.
Weiner also discussed his group’s work developing a DNA-encoded monoclonal antibody (dMAb) platform. Muscle or skin tissue “is transfected and becomes a factory for expression of the protein. The idea is getting [the antibody] secreted into the bloodstream at detectable levels,” said Weiner. They have developed dMAbs targeting Ebola, HIV, and Zika that induce robust antibody expression and viral protection in animal models. For HIV, multiple dMAbs can be delivered at one time, which has been shown to induce broad neutralizing titers against nine HIV subtypes in non-human primates.
Weiner and collaborators are also working to engineer DNA cassettes that encode self-assembling nanoparticles directly in vivo. Nanoparticles targeting HIV showed improved immune responses versus the monomeric form: “It’s dose sparing, it’s much faster seroconversion and much higher titers, and it elicits very good CD8+ T-Cells,” Weiner said.
mRNA Vaccines: A New Era in Vaccinology
Drew Weissman, of the University of Pennsylvania, discussed recent advances in the development of mRNA vaccines for infectious diseases. Why use RNA? In theory, the cost of mRNA production would be much less than that of protein, which requires large-scale cell culture followed by purification that differs for every protein. Weissman’s group developed a platform using nucleoside-modification and purification techniques to optimize mRNA structures that induce high and long-lived translation when delivered within lipid nanoparticles to peripheral sites.
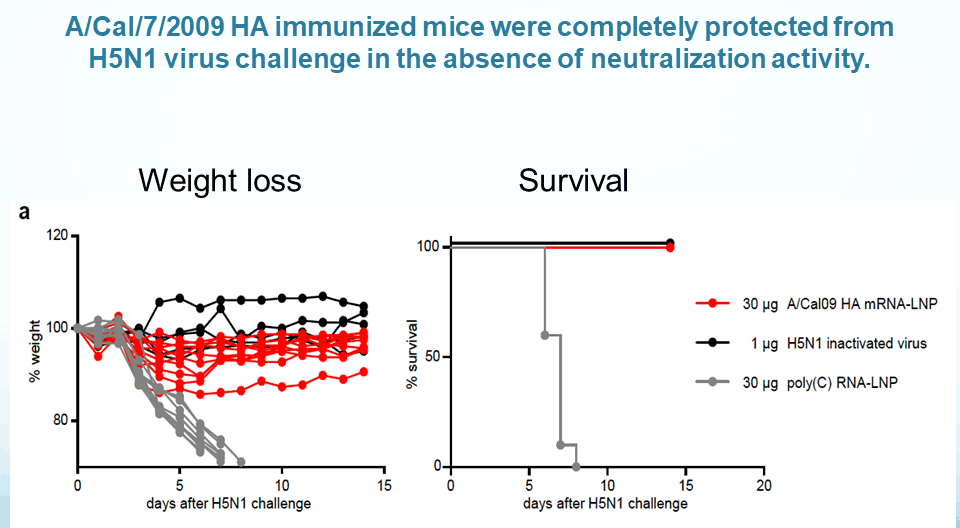
Weissman discussed mRNA vaccines developed with their platform targeting influenza, HSV-2, HIV, and malaria, which have all shown promising results in animal models. For influenza, a single immunization with an mRNA vaccine coding for the hemagglutinin (HA) fusion protein in mice resulted in titers 50 times higher than the current FDA approved vaccine. As a mechanism of action, they found that the lipid nanoparticles used for vaccine delivery induce T- follicular helper cells, which drive long-term immune memory and are “critical in the induction of potent antibody responses,” explained Weissman. Furthermore, their mRNA vaccines induce responses to subdominant epitopes in the presence of dominant epitopes, which isn’t seen with whole proteins. This response is useful because subdominant epitopes, such as the HA stem domain, can be broadly cross-reactive across viral subtypes. Vaccinated mice challenged with distant flu viruses were fully protected, “suggesting that using a full HA could give you a universal vaccine,” said Weissman.
Transforming New Technologies into Vaccines: Genomics, Adjuvants and Self-Amplifying RNAs
Rino Rappuoli, of GlaxoSmithKline, shared how new technologies will allow us to conquer new diseases. Recent advances have allowed for major improvements in reverse vaccinology — using human genomics and structural biology to discover new antigens and instruct vaccine design. “Today we have the tools of synthetic biology,” said Rappuoli. At GSK, “we are using self-amplifying mRNA instead of simple mRNA. We use the replicon of the alphavirus to amplify the RNA and give a better response.” Nucleic acid vaccines work well in animal models, and the challenge now is testing whether it will work well in humans.
Rappuoli also discussed encouraging new advances in antigen delivery using nanoparticles or Generalized Modules for Membrane Antigens (GMMA). While self-assembling natural nanoparticles have been around for years, fully synthetic nanoparticles have only recently been designed. “We are going from mimicking nature to completely computationally designing vaccines,” explained Rappuoli. GMMAs consist of outer membrane vesicles from bacteria, which are engineered to release these vesicles in large quantities with the desired antigens. Rappuoli also highlighted recent developments in adjuvants, substances within vaccines that enhance the immune response to antigens. A promising new adjuvant, AS01, has contributed to breakthrough vaccines for Malaria, tuberculosis, and shingles. Moving forward, Rappuoli aims to use these new technologies to target vaccines for the elderly, emerging infections, and antimicrobial resistance.
Further Readings
Wilson
Impagliazzo A, Milder F, Kuipers H, et al.
A stable trimeric influenza hemagglutinin stem as a broadly protective immunogen.
Science. 2015;349(6254):1301-1306.
Laursen NS, Friesen RHE, Zhu X, et al.
Science. 2018;362(6414):598-602.
Lee PS, Wilson IA.
Structural characterization of viral epitopes recognized by broadly cross-reactive antibodies.
Curr Top Microbiol Immunol. 2015;386:323-341.
Oyen D, Torres JL, Cottrell CA, Richter King C, Wilson IA, Ward AB.
Sci Adv. 2018;4(10):eaau8529.
Oyen D, Torres JL, Wille-Reece U, et al.
Proc Natl Acad Sci U S A. 2017;114(48):E10438-E10445.
Steel J, Lowen AC, Wang TT, et al.
Influenza virus vaccine based on the conserved hemagglutinin stalk domain.
MBio. 2010;1(1).
Turner HL, Pallesen J, Lang S, et al.
PLoS Biol. 2019;17(2):e3000139.
Weiner
Aggarwal C, Cohen RB, Morrow MP, et al.
Clin Cancer Res. 2019;25(1):110-124.
McCoy LE, Burton DR.
Identification and specificity of broadly neutralizing antibodies against HIV.
Immunol Rev. 2017;275(1):11-20.
Patel A, DiGiandomenico A, Keller AE, et al.
Nat Commun. 2017;8(1):637.
Patel A, Park DH, Davis CW, et al.
Cell Rep. 2018;25(7):1982-1993 e1984.
Tebas P, Roberts CC, Muthumani K, et al.
Safety and Immunogenicity of an Anti-Zika Virus DNA Vaccine – Preliminary Report.
N Engl J Med. 2017.
Trimble CL, Morrow MP, Kraynyak KA, et al.
Lancet. 2015;386(10008):2078-2088.
Weissman
Craft JE.
Follicular helper T cells in immunity and systemic autoimmunity.
Nat Rev Rheumatol. 2012;8(6):337-347.
Hook LM, Awasthi S, Dubin J, Flechtner J, Long D, Friedman HM.
Vaccine. 2019;37(4):664-669.
Kaminski DA, Lee FE.
Antibodies against conserved antigens provide opportunities for reform in influenza vaccine design.
Front Immunol. 2011;2:76.
Kariko K, Muramatsu H, Ludwig J, Weissman D.
Nucleic Acids Res. 2011;39(21):e142.
Rappuoli
Garcon N, Chomez P, Van Mechelen M.
GlaxoSmithKline Adjuvant Systems in vaccines: concepts, achievements and perspectives.
Expert Rev Vaccines. 2007;6(5):723-739.
Lal H, Cunningham AL, Godeaux O, et al.
Efficacy of an adjuvanted herpes zoster subunit vaccine in older adults.
N Engl J Med. 2015;372(22):2087-2096.
Marcandalli J, Fiala B, Ols S, et al.
Cell. 2019;176(6):1420-1431 e1417.
Rappuoli R, Aderem A.
A 2020 vision for vaccines against HIV, tuberculosis and malaria.
Nature. 2011;473(7348):463-469.
Rappuoli R, Mandl CW, Black S, De Gregorio E.
Vaccines for the twenty-first century society.
Nat Rev Immunol. 2011;11(12):865-872.