Degradation in Cellular Processes: 2024 Dr. Paul Janssen Award Symposium
Lynne Maquat and Alexander Varshavsky were recognized for their fundamental discoveries in the regulated degradation of RNAs and proteins.
Published April 15, 2025
By Carina Storrs
Academy Contributor
Overview
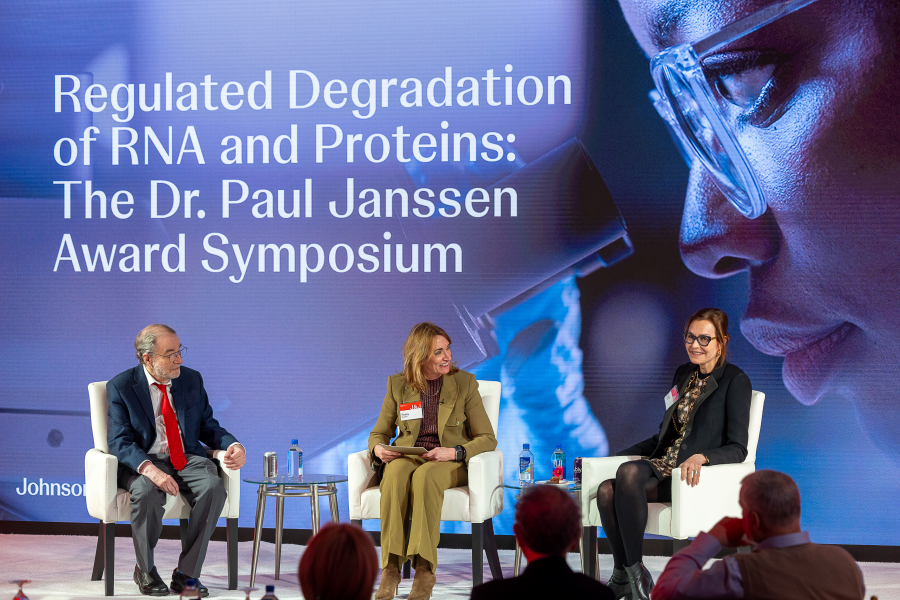
Degradation plays a fundamental role in eliminating abnormal RNA species and misfolded proteins. However, the nonsense-mediated mRNA decay (NMD) and ubiquitin pathways are also central to the regulation of countless cellular processes, from the cell cycle to differentiation and apoptosis.
Lynne Maquat, professor in the Department of Biochemistry and Biophysics at the University of Rochester and Alexander Varshavsky, Thomas Hunt Morgan Professor of Biology at the California Institute of Technology (Caltech), have focused their research careers on defining the molecular players and cellular functions of the NMD and ubiquitin pathways, respectively. Their discoveries have paved the way for new approaches in therapeutic drug development, and in particular methods for destroying proteins, and the messenger RNAs (mRNAs) that encode them, that drive disease states and that have so far been difficult to target.
“Renowned for their groundbreaking contributions to the field of regulated RNA and protein degradation, Professor Maquat and Professor Varshavsky have redefined the frontiers of scientific discovery,” said Nicholas B. Dirks, President and CEO of The New York Academy of Sciences.
On January 30, 2025, leaders from academia and industry gathered at the JW Marriot Essex House in New York City to grant the 2024 Dr. Paul Janssen Award to Maquat and Varshavsky for their fundamental discoveries in the regulated degradation of RNAs and proteins. The award symposium featured award lectures from the honorees and a panel discussion about future directions for their research and drug development implications. It was co-presented by The New York Academy of Sciences and the Dr. Paul Janssen Award, with sponsorship by Johnson & Johnson.
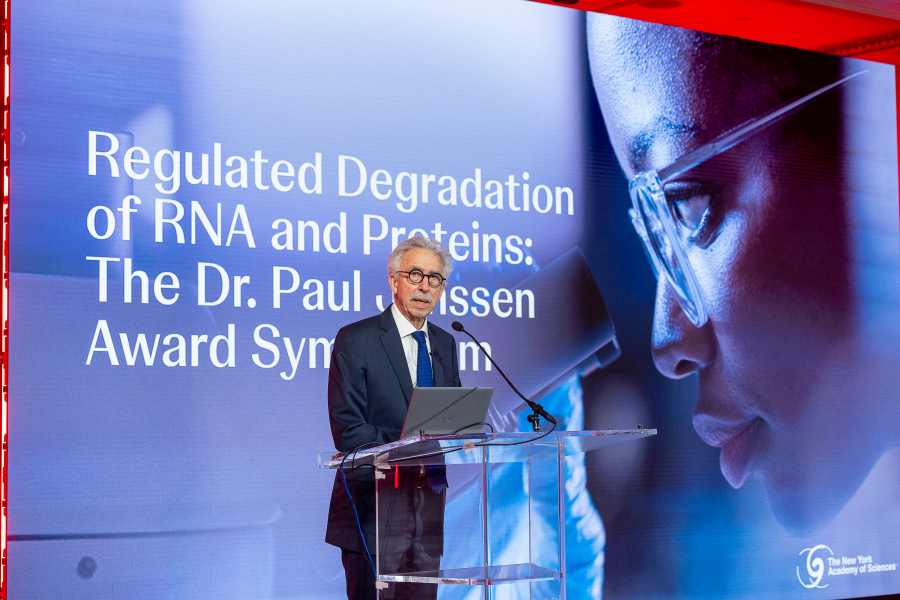
Through videos presented before the award lectures and during the panel discussion, Maquat and Varshavsky shared inspiring stories of how they became interested in science and persevered through challenges. Maquat is the first person in her family to go to college and said an undergrad cell biology class opened her eyes to the fascinating process of protein translation and experimental research. Although Varshavsky grew up surrounded by science, his father was a chemist, he had to defect from the Soviet Union to gain the freedom and resources to realize his potential.
Symposium Highlights
- Nonsense mediated mRNA decay (NMD) plays multiple roles in the cell, including the degradation of both abnormal mRNAs and natural mRNA targets and regulation of cellular adaptive processes.
- The position of introns in genes can modulate the activity of NMD to either enhance or suppress mRNA expression.
- FMRP, the protein missing in Fragile X syndrome, interacts with a protein in the NMD complex and modulates the activity of NMD.
- The ubiquitin system acts on almost every protein in the cell, adding a polyubiquitin chain to these protein substrates that results in their swift degradation by the 26S proteasome.
- A wide range of amino acid residues in various positions can function as degrons, or signals for ubiquitin-mediated degradation.
- Understanding degrons and the molecular components of the ubiquitin system has led to the development of PROTACs and other molecules that target undesirable proteins for destruction.
Nonsense-mediated RNA Decay (NMD)–Key Role in Eliminating Abnormal mRNAs
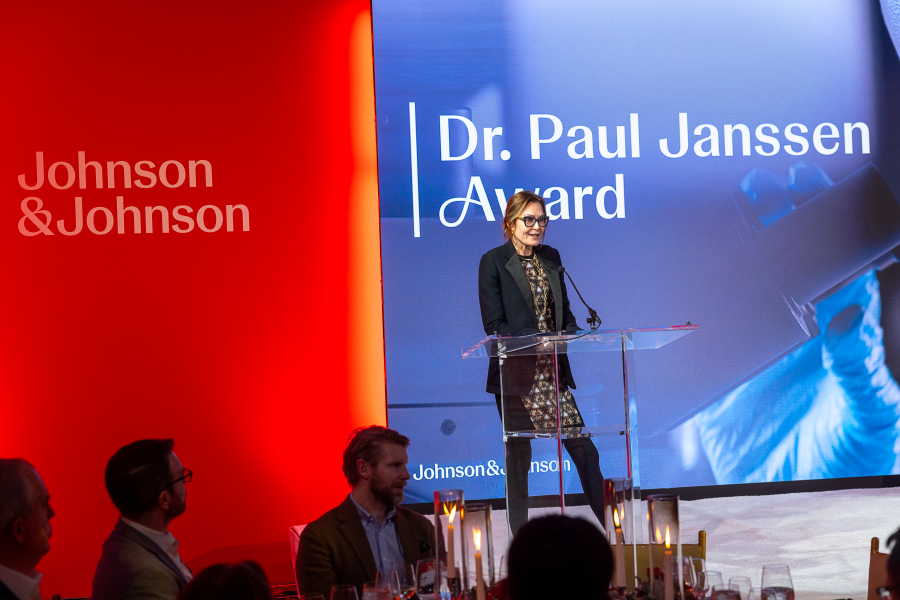
Maquat opened her talk by sharing the intricacies of nonsense-mediated RNA decay (NMD), which she and her lab have spent four decades teasing apart. NMD is a cellular pathway that selectively degrades abnormal messenger RNAs (mRNAs), preventing them from being translated into toxic proteins. It eliminates an estimated one third of mRNAs that acquire mistakes during transcription–specifically frameshift or nonsense mutations that cause premature translation termination and give rise to many diseases, Maquat noted.
As Maquat explained, NMD occurs when the ribosome that translates newly made mRNAs encounters a premature termination codon (PTC) upstream of the normal translation termination (stop) codon. The NMD machinery steps in and chops up the mRNAs. (Maquat noted that an alternative NMD pathway acts on both new and older mRNAs with long or structured 3’ untranslated regions (UTR).)
Many aspects of NMD were still a mystery when Maquat was beginning her career. For one, it was unclear how NMD distinguishes a PTC from a normal stop codon. To their surprise, Maquat and her lab discovered that the positions of introns in pre-mRNA, prior to splicing, signals the presence of a PTC in the processed, spliced mRNA.
Additional experiments allowed Maquat and her lab to describe this relationship at high resolution. They found that PTCs located 50-55 nucleotides or more upstream of the last splice junction trigger NMD, whereas those downstream of this cutoff point do not, in what is known as the “Maquat rule.”
“This is very useful for understanding human diseases. Diseases that are due to a PTC in [the upstream] region are generally recessively inherited, whereas those in the [downstream] region are generally dominantly inherited. This is important for personalized medicine,” Maquat said.
The finding also has important implications for biomedical research. Although putting an intron in a gene can drive up its expression, pharmaceutical companies were putting introns in the 3’ UTR. “We were able to tell them not to do that because you’re triggering NMD, which of course reduces the amount of message,” Maquat said. During the panel discussion that followed the talks, Maquat noted that industry labs embraced her advice, after they saw for themselves that moving introns into the coding regions of cloned genes achieved higher mRNA expression levels.
Another puzzle was that Maquat and her lab were studying the NMD pathway that eliminates mRNAs that are newly made and still associated with the nucleus, but the NMD process relies on translation, which occurs in the cytoplasm. Together with collaborators, Maquat and her lab carried out what she called “killer experiments” using cutting-edge microscopy techniques. By watching mRNAs undergo NMD, they determined that the process acts on mRNAs as they emerge from the nucleus into the cytoplasm. It is rapid, degrading target mRNAs within one minute of entering the cytoplasm. A fraction of NMD targets escape degradation and have a half-life similar to that of mRNAs that are not NMD targets.
Regulation of Stress Responses by NMD
In the second part of her talk, Maquat shared the work she has done to understand a separate, yet also important, function of NMD: cellular adaptation to environmental changes. In addition to abnormal mRNAs, NMD eliminates approximately five to ten percentage points of normal mRNAs, which are targeted due to features such as upstream open reading frames (ORFs) and long 3’ untranslated regions (UTRs).
In one series of experiments, Maquat and her lab treated cancer cells with chemotherapy to induce DNA damage. They found that this type of cellular stress partially suppressed NMD. As a result, pro-apoptotic mMRNAs that are normally targets of NMD could be translated at higher levels and trigger programmed cell death. They also found that treating cancer cells with an NMD inhibitor in addition to chemotherapy enhanced cell death. NMD is involved in the regulation of numerous other stress responses including hypoxia and skeletal muscle differentiation.
Relationship Between NMD and Fragile X Syndrome
In an unexpected turn, Maquat’s efforts to better understand normal cellular functions of NMD led her and her lab to discover that the pathway plays a key part in Fragile X syndrome (FXS), the most prevalent single-gene cause of intellectual disability and autism. “The way we got into this, like much of what my lab does, is not because we intended to study it, but because that’s where our results took us,” Maquat said.
It started because the lab was searching for molecules that help UPF1, a linchpin RNA-binding protein in the NMD machinery. They found that FMRP, the protein missing in FXS, binds to UPF1, and puts the brakes on NMD activity. Without FMRP, NMD is unleashed to degrade thousands of target mRNAs that are present in numerous brain regions, many involved in transmission between neuronal synapses.
Using a mouse model of FXS, in which the gene encoding FMRP is knocked out, Maquat and her lab found that NMD hyperactivity begins during embryogenesis and persists after birth. “We feel like, in mice as a test animal and also in humans, there might be a window of time to dampen the temporary hyperactivated NMD to alleviate some of the [FXS] phenotype,” Maquat said.
In support of this possibility, they observed, through experiments in neurons derived from induced pluripotent stem cells from FXS patients, that treatment with NMD inhibitors helped restore the normal cellular mRNA profile. Maquat and her collaborators are now pursuing drugs that could achieve this effect. (None of the NMD inhibitors they used in their experiments are currently drugs.)
Maquat and her collaborators are also characterizing the functions of FMRP itself, including how it binds to RNA–often at multiple sites, as she shared during the panel discussion–and stalls translation. What they have found bucks the current understanding about several FMRP mechanisms. For example, their experiments revealed that FMRP protects mRNAs from decay by binding their poly(A) tails, via direct binding between FMRP and the poly(A) binding protein PABPC1, along with nonspecific binding to GC-rich regions of mRNA coding sequences.
Pervasive Role of Ubiquitin System in Degradation of Cellular Proteins
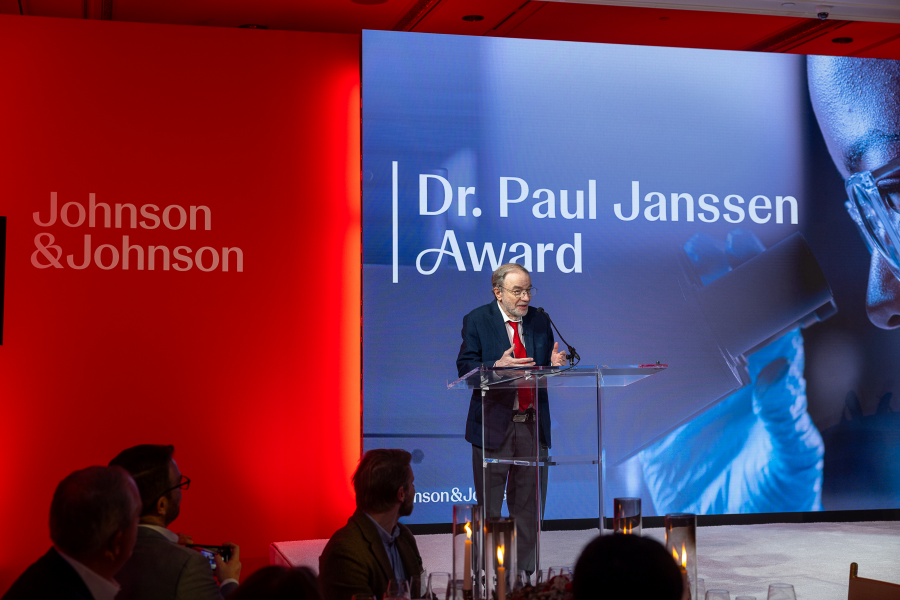
When Varshavsky and his lab, then at the Massachusetts Institute of Technology (MIT), began to study the ubiquitin system in the 1980s, they had no idea of the fundamental cellular role it played, degrading proteins and controlling cellular processes. “But over the next several years, it became clear that the system is unprecedented in its diversity of functions,” Varshavsky said.
Varshavsky began his talk with an overview of the ubiquitin system basics. The pathway begins with the ATP-dependent activation of ubiquitin, a small 76 residue protein, by E1 ubiquitin-activating enzyme. Then ubiquitin is transferred to an E2 ubiquitin-conjugating enzyme. Finally, an E3 ubiquitin ligase transfers ubiquitin to a lysine residue in a substrate protein. “The striking thing is that…just about every single protein in the cell can in principle, and most likely in practice, become a substrate for this reaction,” Varshavsky noted.
The complexity of the ubiquitin system started to reveal itself as Varshavsky’s team found that substrates typically receive not just one ubiquitin molecule but a branched polyubiquitin chain with various topologies. Regardless of the shape they take, polyubiquitin chains direct substrates to the largest protease in the cell, called the 26S proteasome, which rapidly cleaves peptide bonds on proteins as they are threaded through its channel.
By 1986, Varshavsky and his lab identified the first degrons, which are sequences in proteins that tag them for degradation via the ubiquitin pathway. The discoveries kicked off a flurry of activity among pharmaceutical companies to develop PROTACS (proteolysis-targeting chimeras) and PROTAC-like compounds. PROTAC molecules feature a ligand that binds the target protein at one end and a ligand for E3 enzyme on the other end, ideally resulting in the ubiquitination and destabilization of any desired protein.
This research “promises all kinds of advances in medicine that previously were not available because it is very difficult to target proteins for degradation,” Varshavsky noted. Beyond PROTACs, there is a vast amount of research exploring the ubiquitin system, either directly or indirectly. Varshavsky estimates that it accounts for about a quarter of all biomedical research today.
Characterization of Degrons–Molecular Degradation Signals
Varshavsky dedicated much of his talk to a tour of degrons and their molecular pathways. The best understood are N-degrons. Remarkably, as Varshavsky explained, all 20 amino acids can function as a degron when they are the first (N-terminal) residue of a protein if they are positioned in a certain way. Specifically, the amino acid has to be exposed sterically, rather than hidden in a protein fold, and the protein has to harbor a lysine residue to which ubiquitin can be conjugated.
True to the complex nature of the ubiquitin system, there are several N-degron pathways that act on different combinations of N-terminal amino acids. They involve different enzymes that modify the amino acid so it can bind directly to one of various E3 ubiquitin-ligase enzymes, and ultimately undergo efficient degradation by the proteasome. It is a stepwise process that involves a multiprotein complex.
But Varshavsky noted that he and his lab have recently found, through experiments that are not yet published, that once a substrate binds to the complex, some of the downstream steps can be skipped to fast-track N-degron substrates for proteasomal degradation. Varshavsky refers to the phenomenon as superchanneling.
“If you design your N-degrons correctly, and by now we know how to design N-degrons correctly, you can fall from [a full protein] to short peptides in less than 30 seconds,” whether through normal or superchanneling, Varshavsky said.
Although Varshavsky and his lab characterized N-degron pathways over many years through experiments with yeast cells, the system is highly conserved–albeit even more complex–in mice and humans. For example, whereas one of the N-degron pathways in yeast directs substrates to an E3 ubiquitin-ligase enzyme called Ubr1, the counterpart pathway in higher eukaryotes involves Ubr1, Ubr2, Ubr4 and Ubr5. So far, only Ubr1 and Ubr2 have been well characterized. Varshavsky noted that the human genome encodes a total of 600 to 800 E3 enzymes. Moreover, mammalian N-degron pathways can direct substrates not just to proteasomal degradation but also autophagy-mediated destruction.
In addition to N-degrons, degrons inside the protein sequence called internal degrons and C-terminal degron residues or C-degrons can destine proteins for ubiquitin-mediated degradation. Varshavsky did not describe the molecular details of these other degron classes but hinted at their importance by noting that one of the N-degron pathways probably accounts for only 10% to 15% of the entire ubiquitin system.
Interplay Between Ubiquitin System and Countless Cellular Processes
The functions of the ubiquitin system are so widespread as to affect every single cellular pathway that involves proteins. These include controlling DNA replication, repair, transcription, meiosis, the cytoskeleton, cell differentiation and adaptive and innate immunity. “It kind of gets boring because you basically cite a cell biology textbook,” Varshavsky joked.
In one example, Varshavsky and his lab discovered that yeast cells use the N-degron pathway to regulate the uptake of di and tripeptide nutrients from the environment. When nutrients are scarce, yeast cells don’t waste their energy making high levels of PTR2, the transmembrane pump protein that imports nutrients. A protein called CUP9 blocks the transcription of PTR2, keeping its levels low. However, if peptides become more abundant in the environment, some make their way into the cell, and because they possess N-degrons, bind the E3 ligase UBR1. The association changes UBR1’s conformation and enhances its ability to degrade CUP9, which in turn boosts the level of PTR2 to uptake more nutrients.
Another critical cellular of the N-degron pathway is apoptosis. As cells enter the programmed cell death pathway, many proteins are cleaved by caspases and other enzymes and the remaining fragments are pro-apoptotic. Varshavsky and his lab found that these fragments are also N-degron substrates, and by rapidly degrading them, the N-degron pathway actually reins in apoptosis and keeps cells from dying too easily, such as to protect against neurodegeneration.
Panel Discussion: Where We Go from Here
- Discoveries made by Maquat and Varshavsky and their labs have contributed to the development of therapeutic candidates that act on RNA and protein degradation pathways.
- Future research should help define these pathways at a molecular level in order to help understand potential off-target effects and develop more specific drugs.
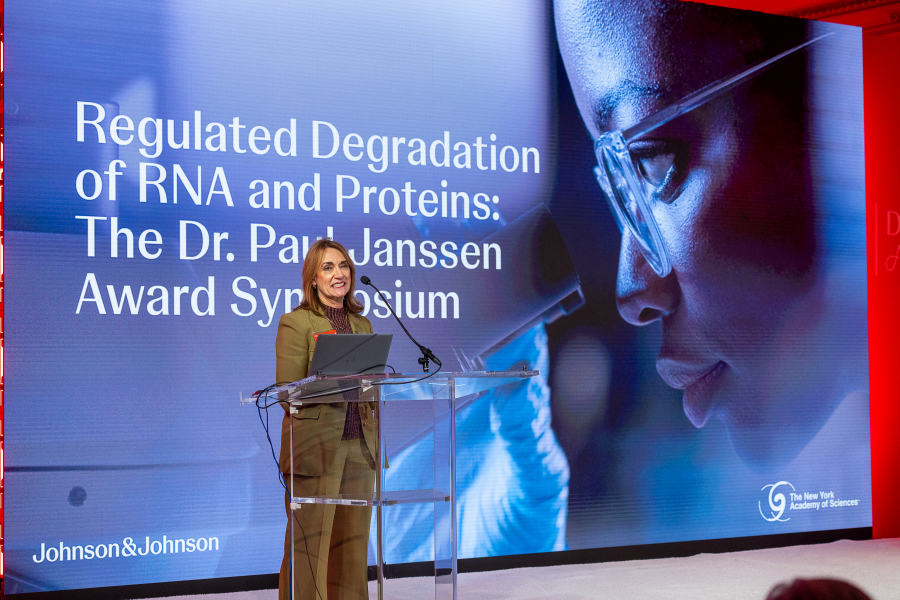
The day closed with a panel discussion in which Maquat and Varshavsky responded to questions from moderator Penny Heaton and the audience. The conversation spanned topics from therapeutic implications of the data that were presented to new research directions, approaches and technologies.
To help open the discussion, Heaton asked what each of the honorees would like to see happen next in their work. Maquat hopes to identify a small molecule drug to treat Fragile X syndrome. Toward this end, she and her lab are working with chemists and a pair of biotech companies to develop these compounds and test them in FXS mouse models. Maquat has funding from the FRAXA Research Foundation, and would like to move a small molecule into clinical studies. “It’s very rewarding to see companies enacting some of the work we’ve done in drug development,” Maquat said.
For his part, Varshavsky shared a question that has fascinated him since he was an undergrad student at Moscow University: why do we—and probably all eukaryotes—sleep? As he described, “sleep is a bad idea.” It makes an individual vulnerable to predation, attack, and other undesirable events. Varshavsky and his lab are pursuing experimentally the molecular reasons for sleep. He specifically wants to explore whether physical inactivity gives N-degron and other degradation pathways a chance to clear the many protein fragments that are constantly generated and thus help protein complexes dissociate and terminate a cell signaling pathway for example.
Audience members inquired about how to untangle the complexities of the protein and mRNA degradation pathways to advance development of drugs that manipulate these pathways. Varshavsky suggested that it may not be critical to understand all the details, such as the structures of polyubiquitin chain, because PROTAC and PROTAC-like compounds that are in development may be effective regardless of these structures.
Maquat mentioned approaches for targeting the mRNAs that encode undesirable proteins, rather than the protein itself. She mentioned work at biotech companies to develop small interfering RNAs (siRNAs) and antisense oligonucleotides (ASOs) that target structures in the mRNA of proteins like those involved in Huntington’s disease. Another approach is to target AKT, a kinase that Maquat and her team found phosphorylates UPF1 in a step that is critical for its NMD activity. An oral AKT inhibitor, capivasertib, is already on the market for patients with certain types of metastatic breast cancer.
The discussion touched on the issue of off-target effects. Maquat noted that AKT, along with other UPF1 phosphorylating kinases, have multiple functions in the cells. The risk of altering or losing these functions will have to be weighed against the benefit of eliminating an undesirable function. She also mentioned that NMD activity varies based on the mRNA species, cell type and development stage. For example, immunoglobulin genes produce NMD targets at high frequency, and pluripotent cells have more efficient NMD than differentiated ones.
Heaton inquired whether the honorees use generative AI and what role they see for this technology. In Maquat’s experience, it is not ready for primetime. She and her team have not been able to confirm interactions predicted by AI, although she still hopes for advances that will allow better prediction of RNA structures. Varshavsky noted that AI is in its infancy, and “we shouldn’t even bother to predict” what it will be capable of as it matures.
To conclude, the honorees each shared one change that would improve their collaborations with industry. Maquat suggested more simple material transfer agreements (MTAs)—a goal that Heaton noted Johnson & Johnson is working toward. Varshavsky would like to see industry provide more research grants.
For more information about the Dr. Paul Janssen Awards and to watch video featuring award winners visit: pauljanssenaward.com.
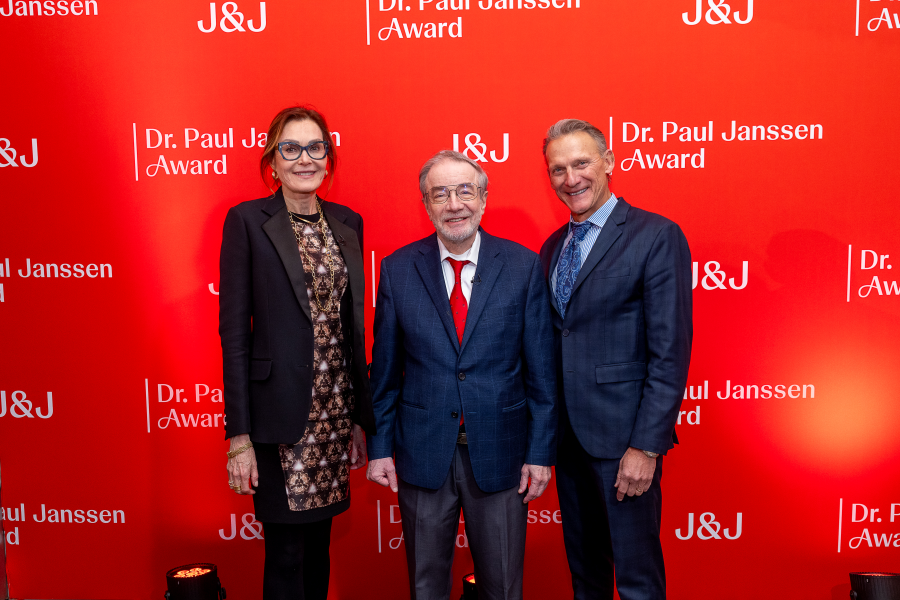