Transformative Research in Neurodegenerative Disease and Neuropsychiatric Disorders: 2017 Innovators in Science Award Symposium
Reported by:
Hallie Kapner
Presented by:
Takeda Pharmaceutical Company Limited
The New York Academy of Sciences
Overview
On November 29, 2017, Takeda Pharmaceutical Company Limited and the New York Academy of Sciences hosted the inaugural Innovators in Science Award Symposium. The event showcased the research accomplishments of the 2017 Innovators in Science Award Honorees in neuroscience and distinguished researchers who are transforming the therapeutic landscape for neurological disease. Topics included advances in optogenetics; microglia and the role of the brain’s innate immunity in diseases including Alzheimer’s disease; advances in designing organoid systems to model complex neurodevelopmental disorders; and efforts to blend big data and systems biology to better understand the genetic drivers and heritability of autism.
Speakers
Michael Halassa, MD, PhD
Massachusetts Institute of Technology
Viviana Gradinaru, PhD
California Institute of Technology
David Julius, PhD
University of California, San Francisco
Frederick Christian Bennett, MD
Stanford University Medical School
Shigetada Nakanishi, MD, PhD
Suntory Foundation for Life Sciences Bioorganic Research Institute
Rudolph E. Tanzi, PhD
Harvard University
Paola Arlotta, PhD
Harvard University
Daniel Geschwind, MD, PhD
University of California, Los Angeles
Sponsors
Early-Career Scientist Award Honoree Lectures
Speakers
Michael Halassa, MD, PhD
Massachusetts Institute of Technology
Viviana Gradinaru, PhD
California Institute of Technology
Highlights
- In conjunction with the prefrontal cortex, the thalamus plays an essential role in forming and applying abstract associations.
- Modulating thalamic activity may have therapeutic benefit in neuropsychiatric disorders such as schizophrenia and autism.
- Advances in optogenetic technologies allow researchers to map long-range pathways in the brain—an important step toward developing new therapies as well as understanding the impact of existing therapies including deep brain stimulation.
- Customized viral vectors capable of delivering actuator genes to the brain via the circulation are a potential means for non-invasive neural modulation.
Rethinking the Thalamus
Michael Halassa opened the Symposium with the first of two presentations from early-career scientists whose findings hold promise as the basis of future therapies.
Halassa’s work challenges conventional beliefs about the role of the thalamus, suggesting that rather than a simple relay for delivering sensory information to the brain’s cortex, the region is a “superhighway” that facilitates the application of abstract associations formed in the prefrontal cortex. The ability to capture information about the outside world, organize and preserve it hierarchically, and apply those associations in real-time form the basis not only of perception, but of higher-order skills such as prediction and storytelling. Halassa explained that dysfunction in these uniquely human mechanisms underlie schizophrenia, autism, and other neuropsychiatric disorders. Understanding the role of the thalamus in these processes may lead to new therapies and novel approaches to cognitive enhancement.
While the thalamus is, indeed, a sensory relay, only a small portion of the structure is responsible for these functions, while most play no role in sensory processing, Halassa explained. The mediodorsal thalamus (MD) is involved in working memory and other cognitive tasks, and is strongly connected to the prefrontal cortex, the seat of executive functioning and decision-making. Experiments in mice reveal that the thalamus is a critical conduit for interaction between sensory information entering the thalamus and abstract thoughts and associations in the prefrontal cortex.
Mice were trained to associate sensory stimuli—in this case, two distinct sounds—with a specific task directive. One sound indicated that the mouse should respond to a visual cue, the other indicated attention to an auditory cue. Using optogenetics to visualize and modulate regions of the thalamus and prefrontal cortex, the researchers discovered that the process of turning a sensory stimulus into a behavioral rule relies on input from the prefrontal cortex. When either the prefrontal cortex or the thalamus is inhibited, mice can no longer direct their attention accordingly— the ability to tap into the association and follow the rule is impaired.
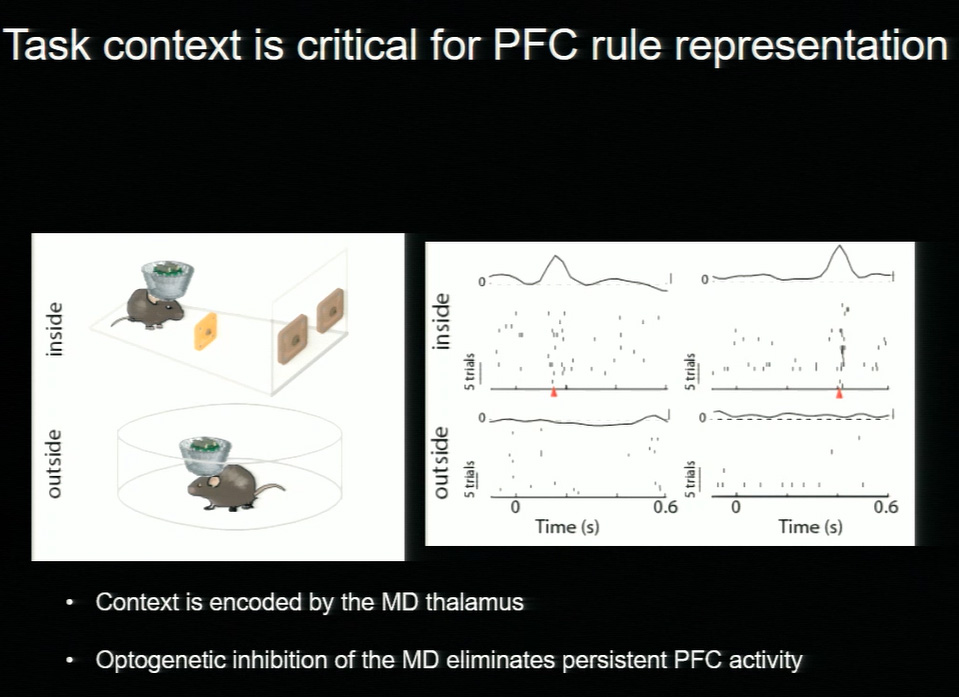
Patterns of neuronal activity show that while the prefrontal cortex is critical for forming associations, the thalamus sustains them. “Signals in the thalamus unlock associations and encode context, which allows the brain to be flexible depending on the circumstances,” said Halassa. When mice were given the same sound cues outside the experimental environment, they make no association. “Different stimuli have different meanings depending on the context, and we see this in everyday life—if you see a red light while you’re driving, you stop. If you see a red light at a dance club, you don’t,” he explained.
Pharmacologic enhancement or dampening of thalamic function may hold promise as a means to address the dysfunctions in contextualization and abstract associations seen in schizophrenia and other psychiatric disorders.
New Approaches to Visualizing and Controlling Brain Circuity
Optogenetic tools have allowed researchers to visualize and control the complex circuitry of the brain. Despite these advances, much remains unknown about the neural paths that influence behavior and brain function in health and disease. Viviana Gradinaru, winner of the Early-Career Scientist Innovators in Science Award, discussed cutting-edge refinements to optogenetic techniques that, combined with modern tissue-clearing methods, enable the type of detailed neural mapping critical to harnessing the brain’s circuitry to treat disease.
Microbial opsins, the light-sensitive proteins that make optogenetics possible, can often be adapted to function in mammalian cells with relative ease. “It’s remarkable, when you think about what a big ask it is for these sequences to function the way we want them to,” said Gradinaru, noting that in their native hosts, such proteins enable entirely different functions, such as locomotion. As optogenetics applications have expanded, however, Gradinaru and others have tweaked the sequences and packaging of these workhorse proteins to increase precision, tolerability, functionality, and to devise new means of delivery.
Gradinaru is developing tools capable of illuminating the brain’s long-range pathways. Mapping the fine projections of the brain poses significant challenges: slicing the tissue can compromise reconstruction of the pathways, and the poor optical properties of lipid-rich brain tissue make imaging difficult. In a significant step toward solving these problems, Gradinaru revived the century-old technique of tissue clearing, adding modern improvements that dissolve light-scattering lipids while locking proteins and nucleic acids in place within a hydrogel matrix that preserves structure. The resulting tissue is both transparent and capable of retaining colored labels. Used in tandem with a new class of optogenetic tools, this tissue-clearing technique enables researchers to track individual axons, even through tightly packed bundles.
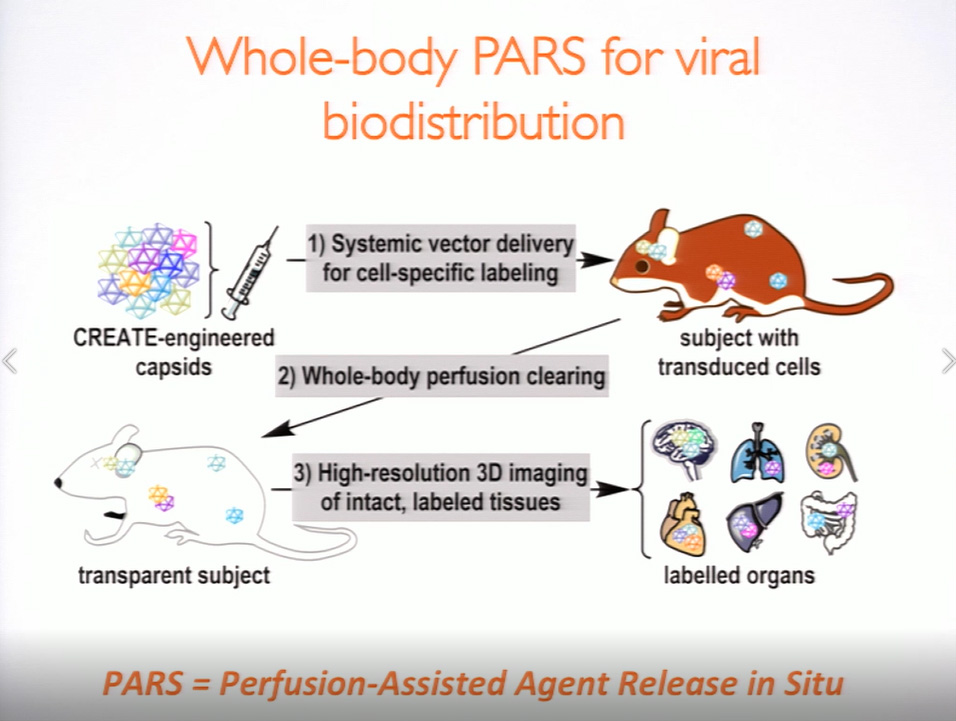
Viral vectors are a common means of introducing labels, sensors, and actuators into the brain, typically via intracranial injection, a method that is invasive and often results in non-homogeneous expression over limited tissue volume. “But what if we could use the vasculature to deliver labels brain-wide, and devise a way to control the density of the labels?” Gradinaru asked, noting that the major hurdle to delivering opsins to the brain is finding a vector capable of circumventing the blood-brain barrier. Using directed evolution, Gradinaru and her team at Caltech engineered a strain of a common viral vector. The adeno-associated virus is capable of passing through the blood-brain barrier and delivering a customized package of protein sequences and gene-regulatory elements that allow researchers to refine a target and restrict expression to certain cell types. It is also possible to limit expression within a cell type, which reduces the density of the label and allows for more precise observations. “We can systemically deliver various colors and direct their expression in the brain, then clear the tissue and reconstruct the morphology to see the long-range projection pathways,” said Gradinaru. Looking to the future, she envisions systemically delivered genes (e.g. actuators or editing tools) as a non-invasive method of modulating neuron biochemistry and activity.
Speaker Presentations
Further Reading
Michael Halassa
Halassa MM, Kastner S.
Thalamic functions in distributed cognitive control.
Nat Neurosci. 2017 Dec;20(12):1669-1679.
Wimmer RD, Schmitt LI, Davidson TJ, et al.
Thalamic control of sensory selection in divided attention.
Nature. 2015 Oct 29;526(7575):705-9.
Wells MF, Wimmer RD, Schmitt LI, et al.
Thalamic reticular impairment underlies attention deficit in Ptchd1(Y/-) mice.
Nature. 2016 Apr 7;532(7597):58-63.
Griffin JD, Fletcher PC.
Predictive processing, source monitoring, and psychosis.
Annu Rev Clin Psychol. 2017 May 8;13:265-289.
Schmitt LI, Wimmer RD, Nakajima M, et al.
Thalamic amplification of cortical connectivity sustains attentional control.
Nature. 2017 May 11;545(7653):219-223.
Viviana Gradinaru
Gradinaru V, Thompson KR, Deisseroth K.
eNpHR: a Natronomonas halorhodopsin enhanced for optogenetic applications.
Brain Cell Biol. 2008 Aug;36(1-4):129-39.
Gradinaru V, Zhang F, Ramakrishnan C, et al.
Molecular and cellular approaches for diversifying and extending optogenetics.
Cell. 2010 Apr 2;141(1):154-165.
Flytzanis NC, Bedbrook CN, Chiu H, et al.
Nat Commun. 2014 Sep 15;5:4894.
Cho JR, Treweek JB, Robinson JE, et al.
Dorsal raphe dopamine neurons modulate arousal and promote wakefulness by salient stimuli.
Neuron. 2017 Jun 21;94(6):1205-1219.e8.
Gradinaru V, Mogri M, Thompson KR, et al.
Optical deconstruction of parkinsonian neural circuitry.
Science. 2009 Apr 17;324(5925):354-9.
Chung K, Wallace J, Kim SY, et al.
Structural and molecular interrogation of intact biological systems.
Nature. 2013 May 16;497(7449):332-7.
Yang B, Treweek JB, Kulkarni RP, et al.
Single-cell phenotyping within transparent intact tissue through whole-body clearing.
Cell. 2014 Aug 14;158(4):945-958.
Treweek JB, Chan KY, Flytzanis NC, et al.
Nat Protoc. 2015 Nov;10(11):1860-1896.
Chan KY, Jang MJ, Yoo BB, et al.
Nat Neurosci. 2017 Aug;20(8):1172-1179.
Deverman BE, Pravdo BL, Simpson BP, et al.
Cre-dependent selection yields AAV variants for widespread gene transfer to the adult brain.
Nat Biotechnol. 2016 Feb; 34(2):204-209
Senior Scientist Award Honoree Lectures
Speakers
David Julius, PhD
University of California, San Francisco
Frederick Christian Bennett, MD
Stanford University Medical School
Shigetada Nakanishi, MD, PhD
Suntory Foundation for Life Sciences Bioorganic Research Institute
Highlights
- Studies of mechanisms by which natural compounds such as capsaicin and menthol modulate temperature-sensitive ion channels in the peripheral nervous system are leading to greater understanding of pain perception.
- Recent experiments reveal that while microglia lose expression of highly specific, signature genes in culture, reintroduction to the CNS environment induces their re-expression and a return to functionality, hinting at the possibility for microglial transplant as a therapy for diseases including Alzheimer’s.
- Discoveries over the past three decades have revolutionized our understanding of neurotransmitter-receptor interactions and the role of these pathways in health and disease.
Probing the Pain Pathways
David Julius began a session of presentations by senior scientist honorees with a discussion of pain pathways—crucial protective mechanisms that often go awry, resulting in prolonged suffering and disability. According to Julius, pain is the primary reason patients seek medical help, and in the United States alone, up to 100 million people suffer some form of persistent pain.
Elucidating the mechanisms of pain—particularly those that drive the shift from acute pain, which is purposeful, to chronic pain, which can persist long after injury subsides—is key to understanding how to address and prevent persistent pain syndromes. A family of cation ion channels, the Transition Receptor Potential or TRP channels, play major roles in sensory physiology, facilitating detection of a wide range of both endogenous and exogenous stimuli including temperature, pressure, chemical irritation, and inflammation. Julius discussed his lab’s discoveries about the structure, functionality, and modulation of a subset of TRP channels tied to temperature and/or chemical sensitivity: TRPV1, TRPM8, and TRPA1.
Julius and his collaborators turned to nature for both inspiration and information to guide their research, focusing on several of the plant kingdom’s natural defense mechanisms: the potent chemicals capsaicin, which produces the sensation of heat in chili peppers; menthol, the cooling agent in mint oils; and thiosulfinates, pungent compounds found in onion and garlic. These “homegrown chemical warfare agents” ward off predators by producing an acute pain response, and it is the impact of these chemicals on TRP channels that form the basis of research that is answering foundational questions about the mechanisms and structure of these complex cellular sensors and their role in pain signaling.
Heat, Cold, Pain, Pressure
Two of the ion channels studied, TRPV1 and TRPM8, are temperature sensors, with the former activated by heat and the latter by cold. Experiments show that TRPV1 can be activated chemically by capsaicin, but also by changes in ambient temperature. “The channel is quiet at body temperature, but at about 43 degrees, it strongly activates,” said Julius. “Interestingly, this is the psychophysical threshold at which most of us discriminate between an innocuously warm object versus one that’s noxiously hot.”
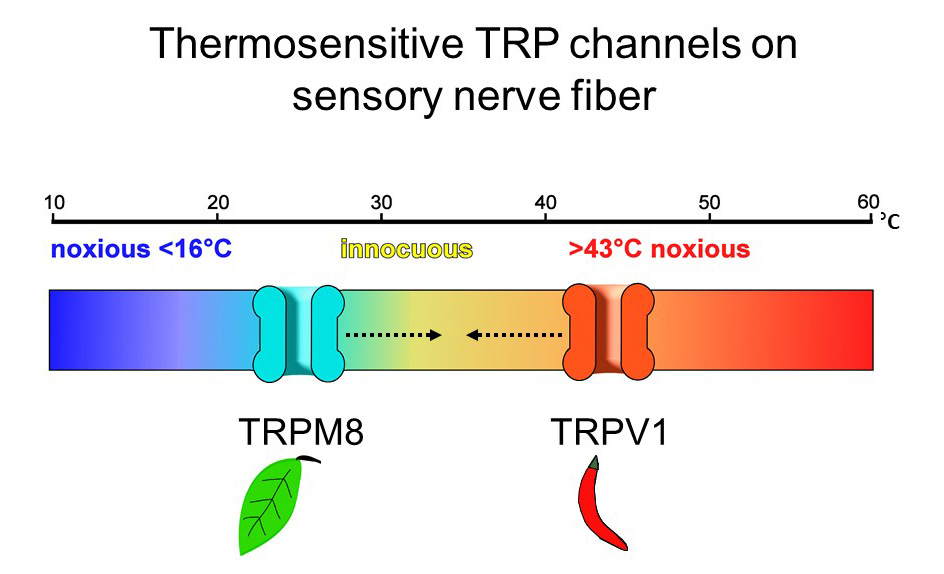
Conversely, TRPM8 is activated at temperatures below 25 degrees, as well as by exposure to menthol. In a physiologic twist, actual sensations of cold and heat and chemical compounds that mimic cold and heat are perceived similarly by the peripheral sensory nervous system. Further studies reinforce that these proteins are intrinsically temperature-sensitive, and serve as molecular thermometers essential for detecting and responding to temperature shifts. To wit: mice bred without a TRPM8 gene are unable to distinguish between a warm compartment and an uncomfortably cold one.
TRPV1 can also be modulated by components of the body’s “inflammatory soup,” explained Julius, noting that while some modulators, including extracellular protons and some lipids, bind directly to the channel and lower its threshold for detecting heat, other modulators, such as prostaglandins and peptides, act on their own receptors yet have the same threshold-lowering effect on TRPV1. “Understanding how these allosteric components directly or indirectly act on the receptor to change its threshold is a step toward developing drugs that interfere with the ability of these modulators to over-sensitize the channel and contribute to persistent pain syndromes,” Julius said.
Modeling the Channel
A lack of high-resolution atomic structural information has hindered researchers’ efforts to fully understand the functionality and modulation of TRP channels. Julius described how cryo-electron microscopy has yielded the first three-dimensional models of TRP channels and allowed researchers to observe the channels’ gating mechanisms in response to multiple stimuli. Imaging reveals that TRP channels have two operable gates which control ion flow, with some modulators acting specifically on one gate or the other. Julius believes this structure contributes to the channels’ sensitivity and efficiency as sensors. “This suggests that part of the reason TRP channels function so beautifully as polymodal signal detectors is that this two-gate mechanism allows for very rich physiologic modulation,” he said.
More recently, Julius and his collaborators have begun to study TRP channels embedded in lipid nanodiscs, which mimic the native environment of the body. Doing so allows them to visualize the precise location where molecules bind to the channel, including toxins and small molecules—an important step in designing next-generation analgesic drugs to modulate the activity of these channels.
Origin, Environment, and Microglial Identity
Frederick Christian Bennett addressed the Symposium on behalf of the late Ben Barres, Senior Scientist Award finalist. Bennett began with an homage to his mentor, highlighting the pioneering neurologist and neuroscientist’s contributions to discovering the dual role of astrocytes—as critical facilitators of synapse formation and functioning in health, and as highly reactive synapse-and neuronal destroyers in certain stress or disease states. “Glial cells and brain function are intimately intertwined in complicated ways we are just beginning to understand, and which hold enormous potential as treatment targets,” Bennett said, transitioning into a discussion of his own research, which also centers on a type of glial cell—microglia.
Microglia are macrophages often referred to as the resident immune cells of the central nervous system. Arising from the yolk sac early in fetal development and quickly sequestered inside the brain, microglia have an origin unlike other hematopoietic cells in the body, which arise in the bone marrow and differentiate throughout the body. Like astrocytes, microglia are dynamic cells capable of changing state based on context and environment, and much the way astrocytes assume a reactive state in diseases such as Alzheimer’s, ALS, and multiple sclerosis, microglia too become dyshomeostatic in disease.
Until recently, in-depth studies of microglial cell function have been complicated by an inability to distinguish them from other macrophages in the brain. However, microglia express a series of signature genes that differ from those expressed by other macrophages. Antibody-based techniques that bind to TMEM119, a protein highly specific to microglia, have yielded new insights into these unique cells. By tracking expression of TMEM119, Bennett is investigating how the CNS environment impacts microglial function and gene expression, and whether microglial cell transplant may be a viable treatment strategy for brain diseases.
Bone marrow transplants are already used to treat certain disorders that impact brain function, with the goal of introducing hematopoietic cells that will infiltrate the brain and differentiate into healthy microglial cells to replace those malfunctioning due to disease or mutation. Yet, studies show that while stem cells do enter the brain and differentiate into cells that are “microglia-like,” they are not true microglia and do not express TMEM119 or other signature microglial genes. The concept of a true microglial cell transplant is one Bennett deems “provocative,” and despite the early stage of the research in animal models, promising.
Bennett’s research shows that the unique ontogeny of microglia, along with elements of the CNS environment, have a profound influence on the cells’ identity and function. Experiments with cultured mouse microglia reveal that these cells lose all expression of signature genes, including TMEM119, when removed from their native environment. Conversely, in a surprising display of plasticity, cultured microglia resume normal gene expression when returned to the CNS. “Signals in the brain are sufficient to sustain, induce, and re-induce microglial identity,” Bennett said. “We didn’t know that it was possible to take cells out of the brain, reintroduce them, and have them recover their homeostatic state.” He explained that such findings may upend notions about the state of microglia in diseases such as Alzheimer’s. For example, it has been posited that in a CNS environment rendered abnormal by disease, microglia may enter an irreversibly neurodegenerative state. The ability of microglial cells to change gene expression and functionality based on their environment suggests that perhaps changes to the CNS in brain disease may restore microglial function.
Understanding Neurotransmitter-Receptor Interactions
Some of the most fundamental insights about the molecular mechanisms that facilitate communication between neurons have emerged from the work of Shigetada Nakanishi. In a career spanning five decades and counting, Nakanishi has discovered and elucidated the roles for multiple families of neurotransmitters, including the ubiquitous excitatory amino acid glutamate, which is critical for neural plasticity, learning, and memory tasks. In excess, glutamate is also implicated in neurodegenerative disorders.
Glutamate receptors are categorized as either ionotropic, meaning they directly and rapidly control ion flow by opening or closing pores in the neuronal cell membrane; or metabotropic, meaning they act on signaling pathways within the nerve cell. These characterizations were discovered using a technique Nakanishi pioneered to circumvent a major hurdle facing early studies of neurotransmitters. Namely, the substances were simple to extract and study, but the receptors, buried deep within cell membranes, eluded extraction and cloning. Nakanishi’s method, which used electrophysiology to measure expression of glutamate receptors in Xenopus oocyte cell membranes, yielded the molecular identities of more than a dozen subtypes of ionotropic glutamate receptors and eight metabotropic subtypes. In the decades that followed, he has demonstrated the role of various receptors in facilitating key brain functions and behaviors, including responding to reward-seeking or aversive stimuli and discriminating between the presence or absence of light.
Nakanishi described experiments targeting the dopamine pathways that control reward-based learning in the basal ganglia to better understand how these two morphologically similar but functionally distinct pathways modulate reward-seeking and aversive reactions. Rewarding or aversive stimuli enter the nucleus accumbens via glutamate transmission, and these inputs are further transmitted along two parallel pathways—the direct pathway, characterized by neurons that express excitatory D1 dopamine receptors, and the indirect pathway, packed with neurons that express inhibitory D2 receptors. “These pathways modulate behavior through dopamine transmission, but in opposite ways,” said Nakanishi.
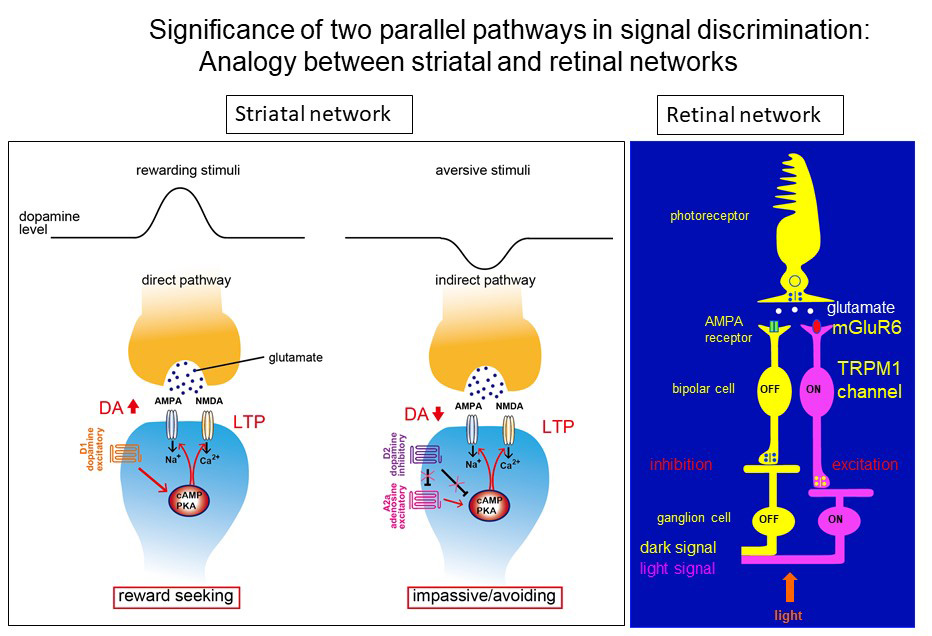
Nakanishi and his collaborators used tetanus toxin to selectively and reversibly block each transmission pathway, discovering that the direct pathway (D1) increases dopamine levels and is responsible for processing rewarding stimuli, while the indirect (D2) pathway processes aversive stimuli and reduces dopamine transmission. More advanced studies of this phenomenon revealed that rewarding and aversive stimuli are converted to activation of excitatory D1 receptors and suppression of inhibitory D2 receptors, respectively; this conversion commonly activates the cAMP-protein kinase A signaling cascade and in turn, stimulates glutamate transmission in a stimulus-dependent, pathway-specific manner.
A similarly structured neural transmission network governs the discrimination between light and dark in the retinal network, explained Nakanishi. Here too, stimulation of highly specific and opposing receptors—AMPA and mGLUR6, both glutamate receptor subtypes—results in the ability to distinguish light and dark signals.
He noted that imbalances in neurotransmitters and functioning of their receptors are implicated in both organic brain diseases and psychiatric diseases, including depression. Illuminating complex neurotransmitter-receptor interactions is critical for understanding healthy brain function as well as the origin and progression of disease, and is fundamental to drug development, as many drugs act directly on receptors.
Speaker Presentations
Further Readings
David Julius
Autzen HE, Myasnikov AG, Campbell MG, et al.
Structure of the human TRPM4 ion channel in a lipid nanodisc.
Science. 2017 Dec 7. pii: eaar4510.
Gao Y, Cao E, Julius D, Cheng Y.
TRPV1 structures in nanodiscs reveal mechanisms of ligand and lipid action.
Nature. 2016 Jun 16;534(7607):347-51.
Liao M, Cao E, Julius D, Cheng Y.
Single particle electron cryo-microscopy of a mammalian ion channel.
Curr Opin Struct Biol. 2014 Aug;27:1-7.
Cao E, Liao M, Cheng Y, Julius D.
TRPV1 structures in distinct conformations reveal activation mechanisms.
Nature. 2013 Dec 5;504(7478):113-8.
Cao E, Cordero-Morales JF, Liu B, et al.
TRPV1 channels are intrinsically heat sensitive and negatively regulated by phosphoinositide lipids.
Neuron. 2013 Feb 20;77(4):667-79.
.
Frederick Christian Bennett
Pfrieger FW, Barres BA.
Synaptic efficacy enhanced by glial cells in vitro.
Science. 1997 Sep 12;277(5332):1684-7.
Christopherson KS, Ullian EM, Stokes CC, et al.
Thrombospondins are astrocyte-secreted proteins that promote CNS synaptogenesis.
Cell. 2005 Feb 11;120(3):421-33.
Stevens B, Allen NJ, Vazquez LE, et al.
The classical complement cascade mediates CNS synapse elimination.
Cell. 2007 Dec 14;131(6):1164-78.
Schafer DP, Lehrman EK, Kautzman AG, et al.
Microglia sculpt postnatal neural circuits in an activity and complement-dependent manner.
Neuron. 2012 May 24;74(4):691-705.
Liddelow SA, Barres BA.
Reactive astrocytes: production, function, and therapeutic potential.
Immunity. 2017 Jun 20;46(6):957-967.
Bennett ML, Bennett FC, Liddelow SA, et al.
New tools for studying microglia in the mouse and human CNS.
Proc Natl Acad Sci U S A. 2016 Mar 22;113(12):E1738-46.
Bohlen CJ, Bennett FC, Tucker AF, et al.
Neuron. 2017 May 17;94(4):759-773.e8.
.
Shigetada Nakanishi
Nakanishi S.
Molecular diversity of glutamate receptors and implications for brain function.
Science. 1992 Oct 23;258(5082):597-603.
Nakanishi S, Hikida T, Yawata S.
Neuroscience. 2014 Dec 12;282:49-59.
Yawata S, Yamaguchi T, Danjo T, et al.
Proc Natl Acad Sci U S A. 2012 Jul 31;109(31):12764-9.
Masu Y, Nakayama K, Tamaki H, et al.
cDNA cloning of bovine substance-K receptor through oocyte expression system.
Nature. 1987 Oct 29; 329(6142):836-8.
Moriyoshi K, Masu M, Ishii T, et al.
Molecular cloning and characterization of the rat NMDA receptor.
Nature. 1991 Nov 7; 354(6348): 31-7.
Masu M, Tanabe Y, Tsuchida K, et al.
Sequence and expression of a metabotropic glutamate receptor.
Nature. 1991 Feb 28; 349 (6312): 760-5.
Masu M, Iwakabe H, Tagawa Y, et al.
Cell. 1995 Mar 10; 80 (5): 757-65.
Hikida T, Kimura K, Wada N, et al.
Neuron. 2010 June 24; 66 (6): 896-907.
Alzheimer’s Disease
Speakers
Rudolph Tanzi
Harvard University
Highlights
- Amyloid deposition begins 10–15 years before patients present with symptoms of Alzheimer’s disease, indicating a need for early identification and treatment of at-risk patients.
- Three-dimensional neural cell cultures that recapitulate Alzheimer’s disease in a dish are facilitating the identification of compounds that prevent or slow the formation of plaques and tangles.
- Beta amyloid is a powerful anti-microbial peptide, and emerging research points to the protein’s immune function as a possible factor in “seeding” plaques that can ultimately lead to Alzheimer’s.
From Rudolph Tanzi’s perspective, the five million patients in the United States with Alzheimer’s are only the tip of the iceberg when it comes to the true scope of the disease. “Plaques begin to form 10–15 years before patients present with symptoms, so that’s potentially 20 or 25 million people who are on the path toward a diagnosis,” said Tanzi, emphasizing that unlike heart disease or cancer, Alzheimer’s disease (AD) requires patients to present with symptoms before treatment begins. “Imagine if we waited until cancer was symptomatic before we treated it?” he said. “Trying to treat plaques once someone has dementia is like trying to put out a forest fire by blowing out the match.”
Tanzi is a co-discoverer of the early-onset familial Alzheimer’s genes, and 30 years after these initial discoveries, the field of genomics has been transformed through advanced sequencing techniques. These techniques allow researchers to identify areas of the genome where AD genes reside as well as mutations that, when studied in mice and in cultured human nerve cells, are leading to next-generation therapeutics aimed at preventing or halting disease progression. Over the past decade, Tanzi and others have built an ever-growing list of more than 200 AD-related mutations impacting one or more aspects of the “trilogy of pathology” underlying Alzheimer’s—amyloid plaques, tangles, and neuroinflammation.
Attempts to recreate Alzheimer’s in mouse models has done little to shed light on the interplay of these factors in causing AD, but in recent years, the advent of brain organoids—human stem cell-derived neural culture systems—has facilitated the creation of what Tanzi terms “Alzheimer’s in a dish.” For the first time, researchers can observe a disease progression that typically takes more than a decade in a matter of weeks. Tanzi reported that by 7 weeks, cells expressing APP or presenilin mutations develop both plaques and tangles, presenting an unprecedented opportunity to test the results of various interventions on the disease process.
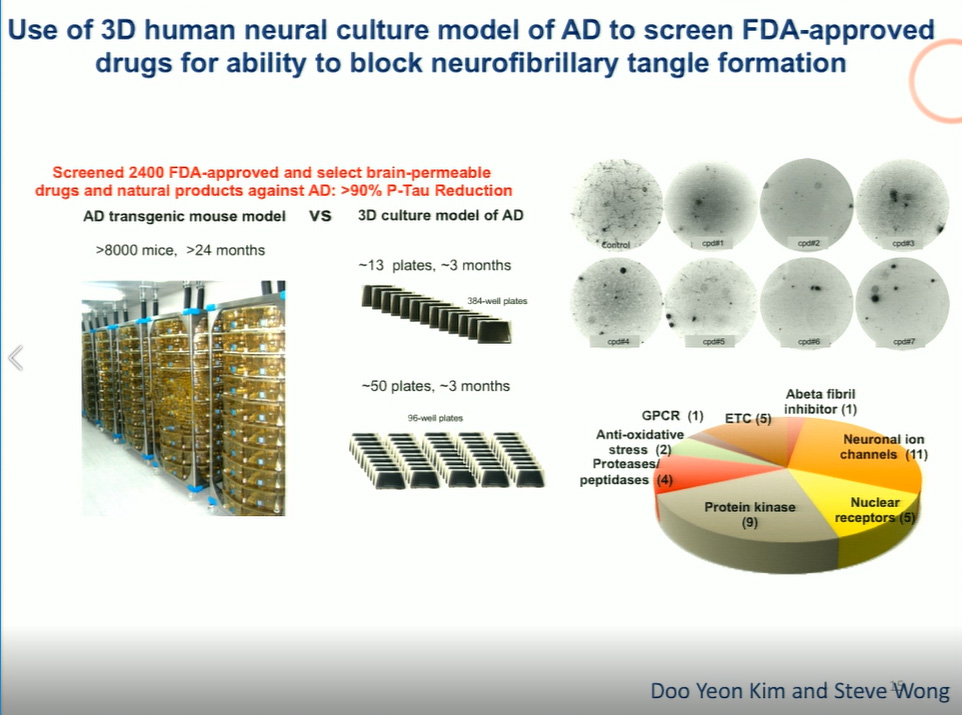
Blocking amyloid deposition through beta or gamma secretase inhibitors successfully reduces the incidence of plaques but also, notably, blocks the formation of tangles—firm proof of the long-debated “amyloid hypothesis,” or the notion that amyloid itself is the driver of AD. Tanzi and his collaborators have also used this model to test compounds that effectively block tangles, identifying 30 FDA-approved drugs that inhibit tangle formation, and another 8 that reduce amyloid deposition, and thus block tangles.
Neuroinflammation is the least-studied factor in AD pathogenesis, yet it warrants greater attention. Tanzi explained that brains that are resilient to Alzheimer’s—those replete with plaques and tangles that never cause dementia—are united by a common feature: a lack of inflammation. “You can have lots of plaque and tangles, but if the brain’s immune system doesn’t react to them with gliosis, you can escape dementia,” he said. Genes including TREM2 and CD33 are implicated in the neuroinflammatory process by regulating the activity of microglial cells. Mutations or knockouts of these genes can impair microglial plaque clearance as well as promote pro-inflammatory changes in these critical immune cells.
Tanzi concluded by describing recent studies that reveal a surprising dual role for beta amyloid, not only as a pathogenic protein in AD but also as an anti-microbial peptide and an essential component of the brain’s innate immunity. The tendency of amyloid beta to form plaques, viewed as inherently pathological in AD, is a powerful protective mechanism when viewed in an immune context. The protein binds to carbohydrates on microbial surfaces, agglutinates, and ultimately traps invading pathogens within a plaque. When HSV1 and Salmonella are introduced into mouse brains, beta amyloid deposition skyrockets, forming plaques in as little as 24 hours. “It’s still early days for this research, but it may be that microbes are seeding amyloid deposition in the brain. This is an entirely different way to look at the origin of Alzheimer’s,” Tanzi said.
Further Readings
Rudolph Tanzi
Goldgaber D, Lerman MI, McBride OW, et al.
Science. 1987 Feb 20;235(4791):877-80.
Kang J, Lemaire HG, Unterbeck A, et al.
The precursor of Alzheimer’s disease amyloid A4 protein resembles a cell-surface receptor.
Nature. 1987 Feb 19-25;325(6106):733-6.
Tanzi RE, Gusella JF, Watkins PC, et al.
Amyloid beta protein gene: cDNA, mRNA distribution, and genetic linkage near the Alzheimer locus.
Science. 1987 Feb 20;235(4791):880-4.
Choi SH, Kim YH, Hebisch M, et al.
A three-dimensional human neural cell culture model of Alzheimer’s disease.
Nature. 2014 Nov 13;515(7526):274-8.
Hardy J, Selkoe DJ.
The amyloid hypothesis of Alzheimer’s disease: progress and problems on the road to therapeutics.
Science. 2002 Jul 19;297(5580):353-6
Wippold FJ 2nd, Cairns N, Vo K, et al.
Neuropathology for the neuroradiologist: plaques and tangles.
AJNR Am J Neuroradiol. 2008 Jan;29(1):18-22. Epub 2007 Oct 9.
Neuropsychiatric Diseases
Speakers
Highlights
- Long-cultured brain organoids differentiate into many cells types, including light-sensitive retinal cells and neurons with dendritic spines.
- Organoids can be used to model highly penetrant single mutations that impact neurodevelopment, and will soon be a viable method for creating models of polygenic disorders in culture.
- Autism is one of the most heterogeneous neurodevelopmental disorders, encompassing a wide range of phenotypes and a large and rapidly growing number of associated genetic mutations.
- Analyses incorporating genetic, transcriptomic, and phenotypic data are revealing patterns of gene expression in autism, as well as linkages to neuropsychiatric disorders including schizophrenia and bipolar disorder.
Building the Brain: From Embryo to Organoid
“How can we model hugely complex diseases like autism, schizophrenia, and other disorders that impact aspects of personality and behavior that are uniquely human?” asked Paola Arlotta, describing the difficulty of unraveling the mysteries of neurodevelopmental disorders. “If we want to look at the impact of the whole genome on disease, we can’t use an animal model—we need a human model,” she said. Arlotta’s lab builds brain organoids—organ models cultured from embryonic or pluripotent stem cells—to understand both normal and atypical brain development in unprecedented detail.
Organoids are a relatively new but promising model for investigating brain development and neurodevelopmental disorders, but until recently, the lifespan of an organoid was measured in weeks. Thanks to modifications in the protocols, Arlotta’s lab is building brain organoids that grow for nine months or longer, maturing and diversifying into an array of cell types and providing new insights into the human neurodevelopmental process.
Much of this insight has been gleaned through single-cell studies of more than 80,000 cells taken from 30 organoids and examined for cell type classification, patterns of gene expression, and physical characteristics. Arlotta and her primary collaborator, postdoctoral fellow Giorgia Quadrato, reported the discovery of a surprising array of cell types, including astrocytes, radial glia, excitatory and inhibitory neurons, retinal cells—including photoreceptor-like cells that respond to light—and progenitors of the cerebral cortex. While little is known about the patterns of gene expression in cells of the fetal human brain, comparisons of the various cell types identified within the organoids with the limited existing data show high correlation between the cells in the organoid and endogenous fetal brain cells. “With no instruction from the outside, these organoids self-organize and mimic the process of human brain development, including differentiating into these various cell lineages,” Arlotta said. “It really speaks to the power of what’s encoded in the genome.”
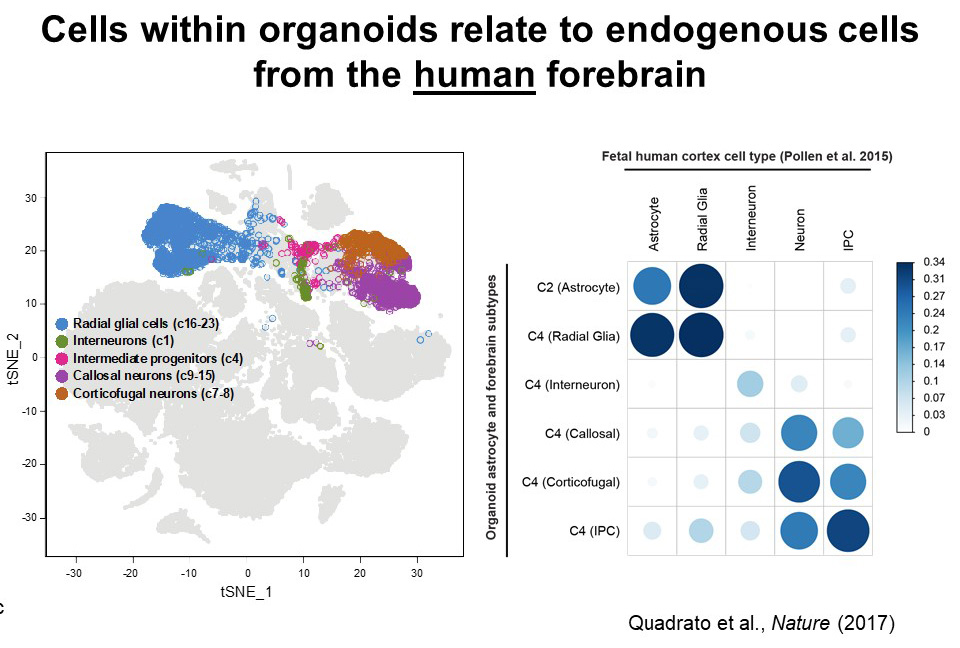
In a subsequent set of findings that stunned both Arlotta and her collaborators, cells from an 8-month-old organoid were shown through electron microscopy to contain dendritic spines, indicating a level of cell maturity difficult to achieve in culture. Psychiatric diseases including schizophrenia are tied to synaptic dysfunction and errors in dendritic pruning, thus the ability to grow cells that form true spines in a dish may present new avenues for exploring genetic drivers of these diseases as well as potential treatments.
“We are heading toward a future where we can engineer highly penetrant mutations into pluripotent stem cells, grow an organoid, and use single-cell sequencing to understand what cell types and pathways are affected by that mutation,” Arlotta said. Noting that most neurodevelopmental diseases are polygenic, she also emphasized the potential for creating chimeric organoids that express different mutations and can be grown from human cells with any genetic background. “An individual’s genomic background is fundamental in controlling the outcome of a mutation, and we’ll soon be able to use organoids to observe how the genome modulates the effect of a certain mutation,” she said.
Autism: Genes, Heritability, and Risk
Daniel Geschwind delivered the final research presentation of the Symposium—a brief but intense dive into efforts to discover the genetics of perhaps the most heterogeneous neurobiological disorder: autism.
Geschwind applies a systems biology approach to the challenge of identifying causal genetics and heritable risk of a disorder that, unlike neurological diseases, evidences no physical manifestations of pathology. Autism is diagnosed based on observations of behavior and evidence of deficits in social interaction and communication, and the disorder encompasses a wide range of phenotypes. Geschwind is building data sets including genetic, transcriptomic, and phenotypic information in an attempt to uncover and understand the linkages between the many manifestations of autism, as well as its connection to other neurodevelopmental and neuropsychiatric disorders.
Geschwind explained that autism is largely driven by common genetic variants, along with some rare de novo mutations, yet no specific gene accounts for more than 1%–2% of cases. A decade ago, fewer than 10 genes were associated with autism, but due to a surge of research interest in the disorder, which affects 1 in 68 children in the United States, more than 200 autism-associated genes have now been identified. Geschwind expects that number to quickly rise to at least 1,000. Due in part to this extreme genetic variability, he and his collaborators are probing the transcriptome in search of patterns of gene expression and regulation that may transcend the genetic and phenotypic heterogeneity of autism.
Comparisons of cortical tissue in autistic and neurotypical brains reveal distinct differences in patterns of co-expression in genes that drive corticogenesis and synaptic function “Most of these genes are expressed at high levels in fetal development, which tells us that a good deal of autism risk that we can identify is occurring during fetal brain development, and that’s quite important,” said Geschwind. Notably, brains of people diagnosed with autism are characterized by downregulation of genes associated with synaptogenesis, and upregulation of genes that regulate microglia and astrocyte activity. As glia are critical for synaptic plasticity, Geschwind believes that this abnormal upregulation of their activity may promote a dyshomeostatic environment that impairs synaptic function in the autistic brain. “It’s also possible that what we see as an upregulation may just be the failure of the neurotypical downregulation of these genes in the first decade of life, so it’s possible that there’s a treatment window there,” Geschwind said.
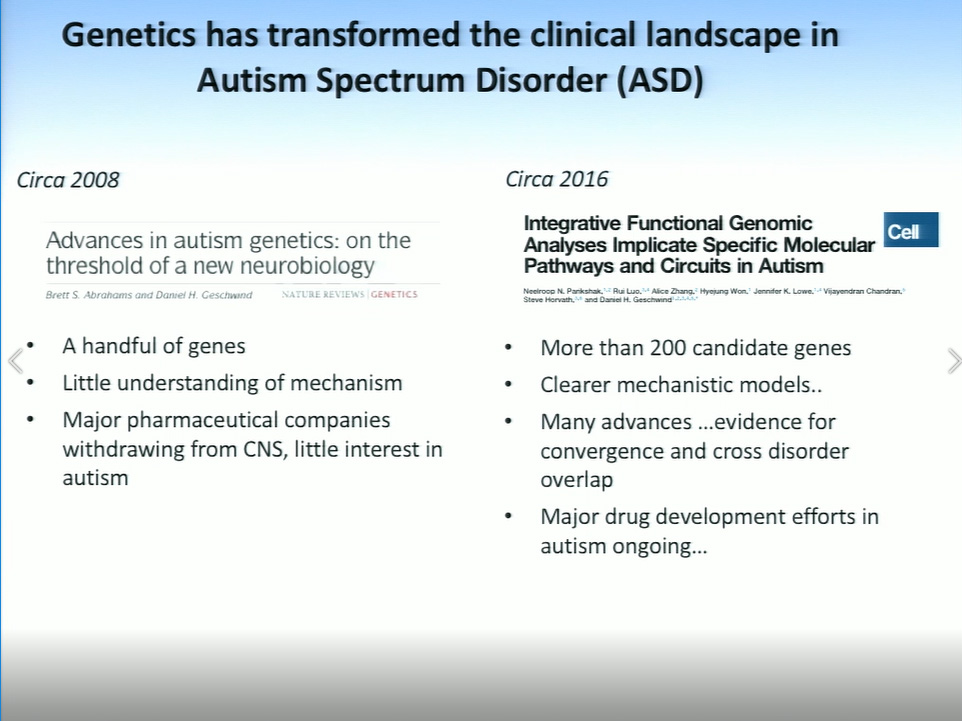
Transcriptomic comparisons between autism and psychiatric disorders including schizophrenia, bipolar disorder, and depression provide additional insights regarding the correlation of disorders that are clinically distinct yet show strong co-heritability. Geschwind described overlaps in gene expression in autism and schizophrenia, as well as in schizophrenia and bipolar disorder—similarities that lay, in part, in the common upregulation of astrocytes and/or microglia in these disorders.
Ascertaining the impact of specific autism-association mutations on neurodevelopment requires a new generation of tools that faithfully recreate in vivo neurodevelopment in vitro or in silico. Geschwind described how machine learning algorithms and 3D neural cell cultures—brain organoids—are advancing the quest to understand the interplay of these complex factors.